Program Value
By almost any measurement, the LDRD program contributes far more in publications, intellectual property, collaborations, and recruitment of postdoctoral researchers—dollar for dollar—than any other program at the Laboratory.
- Collaborative Explorations
- Intellectual Property
- Scientific Publications
- Early Career
- Professional Fellows
- Long-term Impact
Collaborative Explorations
External collaborations are essential to the innovative research that takes place at LLNL, including LDRD-funded projects. By collaborating with other national laboratories, academia, and industry, our investigators can engage with experts from other institutions and access world-class experimental facilities.
The following table provides our most recent data regarding formal collaborations, which we define as LDRD-funded projects where an external collaborator received LDRD funds from LLNL. In addition, our investigators frequently participate in informal collaborations with researchers at other institutions, which often involves joint scientific publications. Both types of collaborations are a key indicator of the broad intellectual engagement that is a hallmark of LLNL’s research environment.
62 institutions were involved in formal collaborations with LLNL as part of LDRD-funded
research teams in FY21
Collaborations | FY17 | FY18 | FY19 | FY20 | FY21 |
---|---|---|---|---|---|
LDRD-funded projects with one or more formal collaborations | 62 | 74 | 74 | 78 | 88 |
Percentage of all projects at LLNL | 29% | 31% | 30% | 32% | 33% |
Scientists focus on cone targets to enhance temperature of electron beams
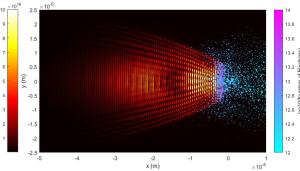
Intense short-pulse laser-driven production of bright high-energy sources, such as x rays, neutrons, and protons, has been shown to be an invaluable tool in the study of high-energy-density science.
To address some of the most challenging applications, such as x-ray radiography of high areal density objects for industrial and national security applications, both the yield and energy of the sources must be increased beyond what has currently been achieved by state-of-the-art high-intensity laser systems.
An LDRD-funded research team at LLNL partnered with the University of Texas at Austin and General Atomics to take on this challenge. Specifically, the team conducted experimental measurements of hot electron production using a short-pulse, high-contrast laser on cone and planar targets.
The cone geometry is a Compound Parabolic Concentrator (CPC) designed to focus the laser to the tip. The cone geometry shows higher hot electron temperatures than planar foils. Simulations identified that the primary source of this temperature enhancement is the intensity increase caused by the CPC.
Andrew Mackinnon, the project’s principal investigator, is using these CPC targets for the project. “These experiments showed that miniature plasma mirror targets do improve coupling of petawatt-class lasers to MeV (mega-electronvolt) electrons, which benefits potential applications such as laser-based MeV radiography,” he said.
The team used the Texas petawatt laser system at the University of Texas at Austin during a six-week period, which has a short pulse and high contrast that allowed the experiment to work. The target is a compound CPC that is specifically designed to focus more laser energy on the tip and increase the intensity.
The Department of Energy’s Office of Science supported the LaserNetUS initiative at Texas Petawatt and LLNL’s Laboratory Directed Research and Development program funded the team and the crucially important target development from General Atomics.
The team has been awarded additional time through LaserNetUS at the Texas petawatt to continue research on CPCs targets, concentrating on the acceleration of the protons from the rear surface and the enhancement that the CPCs provide.
LDRD Project Title: LDRD Project Title: Establishing a Laser-Driven Megaelectronvolt X-Ray and Neutron Radiographic Capability
Principal Investigator:
Andrew Mackinnon
LDRD Project:
19-SI-002
Compact High Efficiency Electrically Tunable Amplifier (CHEETAh)
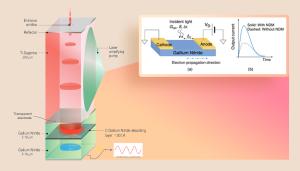
An LDRD-funded team of engineers at LLNL designed a new kind of laser-driven semiconductor switch that can theoretically achieve higher speeds at higher voltages than existing photoconductive devices. The development of such a device could enable next-generation satellite communication systems capable of transferring more data at a faster rate, and over longer distances, according to the research team.
Scientists at LLNL and the University of Illinois Urbana-Champaign (UIUC) reported on the design and simulation of the novel photoconductive device in a paper published in the IEEE Journal of the Electron Devices Society. The device utilizes a high-powered laser to generate an electron charge cloud in the base material gallium nitride while under extreme electric fields.
Unlike normal semiconductors, in which electrons move faster as the applied electrical field is increased, gallium nitride expresses a phenomenon called negative differential mobility, where the generated electron cloud doesn’t disperse, but slows down at the front of the cloud. This allows the device to create extremely fast pulses and high voltage signals at frequencies approaching one terahertz when exposed to electromagnetic radiation, researchers said.
Funded by the LDRD program, the project aims to demonstrate a conduction device that can operate at 100 GHz and at a high power. Future work will examine the impact of heating from the laser on the electron charge cloud, as well as improving understanding of the device’s operation under an electrical–optical simulation framework.
“The goal of this project is to build a device that is significantly more powerful than existing technology but also can operate at very high frequencies,” said LLNL engineer and project principal investigator Lars Voss. “It works in a unique mode, where the output pulse can actually be shorter in time than the input pulse of the laser—almost like a compression device. You can compress an optical input into an electrical output, so it lets you potentially generate extremely high speed and very high-power radio frequency waveforms.”
“If the photoconductive switch modeled in the paper could be realized, it could be miniaturized and incorporated into satellites to enable communication systems beyond 5G, potentially transferring more data at a faster rate and over longer distances,” Voss said. To further optimize performance, researchers are exploring other materials for testing.
LDRD Project Title: Compact High Efficiency Electrically Tunable Amplifier (CHEETAh)
Principal Investigator:
Lars Voss
LDRD Project:
19-DR-015
Intellectual Property
Year after year, projects sponsored by LDRD achieve a disproportionately large percentage of the patents and copyrights issued for LLNL research. As illustrated in the following tables, in recent years, LDRD-funded work has been key in developing more than half of the Laboratory’s patents, one-third of the Laboratory’s copyrights (chiefly computer code), and more than half of the Laboratory’s records of invention.
Patents | FY17 | FY18 | FY19 | FY20 | FY21 |
---|---|---|---|---|---|
All LLNL patents | 88 | 79 | 143 | 200 | 166 |
LDRD patents | 55 | 41 | 95 | 131 | 96 |
LDRD patents as a percentage of total | 63% | 52% | 66% | 66% | 58% |
Copyrights | FY17 | FY18 | FY19 | FY20 | FY21 |
---|---|---|---|---|---|
All LLNL copyrights | 105 | 105 | 118 | 138 | 125 |
LDRD copyrights | 19 | 23 | 24 | 31 | 42 |
LDRD copyrights as a percentage of total | 18% | 22% | 20% | 22% | 34% |
Records of Invention | FY17 | FY18 | FY19 | FY20 | FY21 |
---|---|---|---|---|---|
All LLNL records | 110 | 105 | 129 | 126 | 89 |
LDRD records | 53 | 47 | 65 | 56 | 53 |
LDRD records as a percentage of total | 48% | 45% | 50% | 44% | 60% |
Building on LDRD research to create groundbreaking 3D metal-printing technology
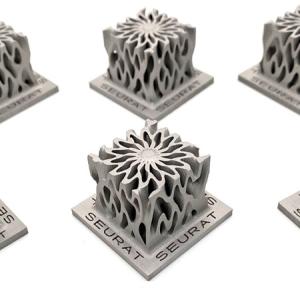
Developed by an industrial partnership between LLNL and Seurat Technologies Inc. of Wilmington, Massachusetts, the technology, called Large-Area Pulsed Laser Powder Bed Fusion (LAPBF) Area Printing™, has the potential to revolutionize metal additive manufacturing (AM). Companies requiring larger metal parts, such as aerospace and automotive manufacturers, are among the industries that could benefit from industrial metal printers with unparalleled speed and resolution.
The technologies enabling Area Printing were developed by Seurat’s CEO, former LLNL researcher James DeMuth, and several LLNL colleagues—some of whom have also gone on to work at Seurat. The company derives its name from the post-impressionist painter Georges Seurat, who studied the science of light and pioneered the painting style known as pointillism.
The path that took DeMuth from a master’s degree in engineering to the verge of launching a groundbreaking new manufacturing technology led through two crucial LDRD projects. After DeMuth joined LLNL and helped design the reaction chamber for an inertial fusion energy (IFE) powerplant, he and his colleagues determined that the only material able to handle the 600°C (1,100°F) heat and rapid temperature fluctuations without cracking was a steel-nanoparticle composite able to maintain its strength at high temperatures.
The researchers concluded that the only process capable of producing the specialized parts for the reactor was a type of additive manufacturing called laser powder bed fusion (L-PBF). Also known as selective laser melting, L-PBF works by shining a laser onto a thin layer of metal powder; the intense laser spot melts the powder and welds it to the layer below.
In 2013, LLNL’s Directed Research and Development (LDRD) program funded a strategic initiative to develop diode-based additive manufacturing (DiAM), an L-PBF technique that can flash-print an entire layer of metal powder at a time. The DiAM research was led by Chris Spadaccini, Bassem El-Dasher, and DeMuth. Using high-powered arrays of laser diodes, a Q-switched (pulsed) laser, and the optically addressable light valve (OALV), DiAM was able to 3D-print metal objects faster than ever before.
A follow-on LDRD program led by LLNL scientist Ibo Matthews funded wide-area photolithographic printing research. The Lab’s Gabe Guss and Reggie Drachenberg played a central role in producing the parts for the project, with contributions from Josh Kuntz and Eric Duoss.
DeMuth recognized that the information gained in the LDRD projects had broad applications to industry. The ability to design and produce additively manufactured metal parts with tailored microstructures that meet stringent performance requirements were crucial.
“This system demonstrated that we could project laser light down to a bed of metal powder, weld a patterned area in an instant, and build a multi-layer part using this technique,” DeMuth writes. “This system architecture was the solution, opening endless possibilities for additive manufacturing.”
LDRD Project Titles: A New Science-Based Paradigm Enabling Microstructure-Tailored Additive Manufacturing of Metals
Principal Investigator:
Manyalibo Matthews
LDRD Project:
18-SI-003
Scientific Publications
Laboratory scientists and engineers publish more than a thousand papers each year in a wide range of peer-reviewed journals, of which LDRD-funded work accounts for a large portion. The numerous publications made possible through LDRD-sponsored research help the Laboratory maintain a strong presence in the broader scientific community, extending the impact of LDRD research beyond the DOE mission space into the wider scientific arena. In addition, the impact of these publications documenting LDRD project results extends long after articles appear in the journals, increasing the value of LDRD investments in these projects.
Journal Articles | FY17 | FY18 | FY19 | FY20 | FY21 |
---|---|---|---|---|---|
All LLNL articles | 1,126 | 1,178 | 1,281 | 1,149 | 1,256 |
LDRD articles | 274 | 456 | 553 | 428 | 509 |
LDRD articles as a percentage of total | 24% | 39% | 43% | 37% | 41% |
LDRD Research Featured in Peer-reviewed Journals
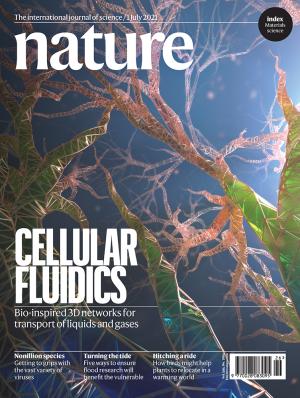
Inspired by the way plants absorb and distribute water and nutrients, LLNL researchers developed a groundbreaking method for transporting liquids and gases using 3D-printed lattice design and capillary action phenomena. In a paper published in Nature and featured on the publication’s cover, LLNL researchers describe 3D-printed micro-architected structures capable of containing and flowing fluids to create extensive and controlled contacts between liquids and gases. The ordered, porous, and open-cell structures facilitate surface tension-driven capillary action (the movement of liquid though small pores due to adhesion and cohesion forces) in the unit cells—akin to a tree pulling water from soil or a paper towel soaking up a spill—and enable liquid and gas transport throughout the structures.
“The problem with these complex environments is that we haven’t had a good way to create model systems to facilitate understanding the fundamental science. For example, we can’t yet make artificial lungs, where you have this complexity of having gases, liquids, and solids co-present,” said Eric Duoss, director of LLNL’s Center for Engineered Materials and Manufacturing and LDRD principal investigator. “But now what we have is a platform to do those fundamental studies that are so important for creating understanding. With that newfound understanding in hand, we will have an incredible opportunity to apply it.”
LDRD Project Title: Manufacturing Molecules for the New Carbon Economy
Principal Investigator:
Eric Duoss
LDRD Project:
19-SI-005
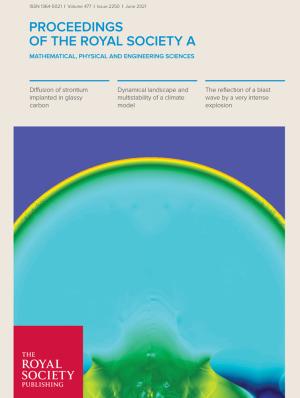
An LDRD project team has taken a closer look at how nuclear weapon blasts close to the Earth’s surface create complications in their effects and apparent yields. Attempts to correlate data from events with low heights of burst revealed a need to improve the theoretical treatment of strong blast waves rebounding from hard surfaces.
This led to an extension of the fundamental theory of strong shocks in the atmosphere, which was first developed by G.I. Taylor in the 1940s. The work represents an improvement to the Lab team’s basic understanding of nuclear weapon effects for near-surface detonations. The results indicate that the shock wave produced by a nuclear detonation continues to follow a fundamental scaling law when reflected from a surface, which enables the team to predict detonation-related damage more accurately in a variety of situations.
The findings, featured in Proceedings A of the Royal Society Publishing, are authored by Andy Cook, Joe Bauer, and Greg Spriggs. The work, “The Reflection of a Blast Wave by a Very Intense Explosion,” was also highlighted on the cover of the publication.
LDRD Project Title: Identifying the Influence of Environmental Effects on Post-Detonation Chemistry and Debris Formation
Principal Investigator:
Kimberly Knight
LDRD Project:
20-SI-006
Early Career Opportunities: Students and Postdoctoral Fellows
By funding exciting, potentially high-payoff projects at the frontiers of science, the LDRD program attracts top talent in new and emerging fields of science and technology. As shown in the following tables, LDRD investments contribute to the health and robustness of LLNL’s student and postdoctoral researcher programs.
Students | FY17 | FY18 | FY19 | FY20 | FY21 |
---|---|---|---|---|---|
Students supported by LDRD | 127 | 138 | 160 | 101 | 136 |
Percentage of all students | 22% | 22% | 23% | 18% | 24% |
Postdoctoral Researchers | FY17 | FY18 | FY19 | FY20 | FY21 |
---|---|---|---|---|---|
Postdoctoral researchers supported by LDRD ≥10% of their time | 137 | 167 | 170 | 208 | 208 |
Percentage of all postdoctoral researchers | 44% | 54% | 57% | 63% | 54% |
LDRD postdoctoral researchers converted to full staff | 31 | 52 | 46 | 60 | 50 |
Percentage of all conversions | 53% | 71% | 68% | 77% | 81% |
Summer students and postdoctoral researchers collaborate on direct drug delivery with carbon nanotube porins
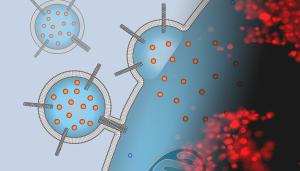
Modern medicine relies on an extensive arsenal of drugs to combat deadly diseases such as pneumonia, tuberculosis, HIV-AIDS, and malaria. But getting those drugs into disease-ridden cells has remained a major challenge for modern pharmacology and medicine. To address this pressing need, career scientists, postdoctoral researchers, and summer students from LLNL, the University of California Merced, and the Max Planck Institute of Biophysics collaborated on the use of carbon nanotubes to enable direct drug delivery from liposomes through the plasma membrane into the cell interior by facilitating fusion of the carrier membrane with the cell.
Drugs are often poorly soluble, strongly toxic to other tissues, or face rapid degradation in the different chemical environments in an organism. They can accumulate in non-target tissues, bind to other cellular components, or may not internalize efficiently into the target cells.
Liposomal delivery systems aim to mitigate these problems by encapsulating drugs in external carriers that circulate through the bloodstream. However, these systems involve a trade-off between enhancing liposomal stability on the way to the target and easing payload release into the cytosol of the target cell.
“We thought that carbon nanotube porins—short pieces of carbon nanotubes inserted into lipid membranes—can mimic viral fusion peptide functionality and help to fuse the liposomal carriers to the membranes of cancer cells,” said scientist Aleksandr Noy, who led the team of LLNL researchers.
In a series of experiments, the team demonstrated that a simple nanomaterial platform—a dimer of small-diameter carbon nanotube porins (CNTPs)—functions as a potent promoter of membrane fusion. Moreover, when Noy and his team loaded their liposomes with a potent chemotherapeutic agent (doxorubicin), these carriers delivered the drug to cancer cells, killing most of them.
“Our results open an avenue for simple and efficient drug delivery carriers compatible with a wide range of therapeutics,” said Nga Ho, LLNL postdoctoral researcher.
Coarse-grained molecular dynamic simulations, performed by the team at Max Planck, revealed a distinct and unusual fusion mechanism where CNTP dimers tether the vesicles, pull the membranes into proximity, and then fuse their outer and inner leaflets.
“We were very happy to see that membrane fusion facilitated by small diameter carbon nanotube porins can lead to complete mixing of the membrane material and vesicle interior content,” said Marc Siggel, a graduate student at Max Planck. Collaborating across institutions and career stages, the co-researchers on this LDRD-funded project understood the potential significance of their work. With new ways to bypass the endocytic pathway, an improved drug delivery strategy could open new doors to therapeutic treatments.
LDRD Project Title: Longitudinal Monitoring of Ribonucleic Acid Content of a Live Cell with a Nanotube Pore Interface
Principal Investigator:
Aleksandr Noy
LDRD Project:
18-ERD-011
Professional Fellows
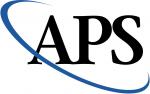
One relevant indicator of advancement and leadership in a scientific field is the election of individuals as fellows of professional societies. This indicator reflects success for both the individual researcher and the Laboratory as a whole.
American Physical Society (APS) fellowships are awarded based on scientific merit and impact over an extended period, and the evaluation process relies on nomination and recommendation by peers. As such, data regarding the history of APS fellowships awarded to LLNL physicists provide an important indicator regarding the key role that the LDRD Program plays in developing the technical, scientific, and leadership skills of early career staff. As presented in the following table, for fiscal year 2021, 100% of the new APS Fellows from LLNL have early career LDRD experience.
Because the quantity of awards each year is a small number, we also present multi-year statistics. For example, over the last 20 years, more than 90% of the APS Fellows at LLNL had early career LDRD experience.
Single-Year Statistics | Multi-Year Statistics | |||||
---|---|---|---|---|---|---|
History of APS Fellows at LLNL | FY19 | FY20 | FY21 | FY11–15 (5 yrs) |
FY16–20 (5 yrs) |
FY00–21 (21 yrs) |
Total APS awards | 6 | 4 | 3 | 25 | 21 | 97 |
Awards with LDRD roots | 5 | 4 | 3 | 24 | 20 | 90 |
% with LDRD roots | 83% | 100% | 100% | 96% | 95% | 93% |
Average years from first LDRD experience | 15.6 | 20.8 | 17 | 13.6 | 18.2 | 12.8 |
Tracing impact to LDRD roots
Throughout this section, we mention “LDRD roots.” Much discussion with principal investigators has transpired about what it means for an accomplishment to have LDRD roots. A simple case would be if an idea for an invention arises during an LDRD project and work on the invention is completed during the period of LDRD investment. But R&D often does not advance on such a short timescale. In general, an accomplishment (invention, paper, capability, etc.) is determined to have LDRD roots if at least one LDRD project needed to occur for the accomplishment to take place. In other words, if one can identify an LDRD project that was critical to the accomplishment, then it is considered to have LDRD roots.
2021 APS Fellows at LLNL
Three LLNL scientists were selected as 2021 fellows of the American Physical Society. The new fellows represent a selection of physics expertise, ranging from intense laser–matter interactions and inertial fusion energy science to leading the development of edge simulation models and codes to pioneering new regimes of warm dense matter experimental science.
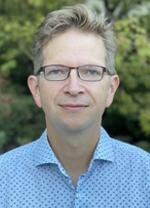
Tilo Doeppner
“I am incredibly honored and grateful to be selected as an APS fellow. It is humbling to be recognized by my peers in the plasma physics community.”
Tilo Doeppner, a physicist in the High Energy Density Division of the National Ignition Facility & Photon Science (NIF&PS) Directorate, was chosen for “pioneering new regimes of warm dense matter experimental science from megabar to gigabar pressures on high-energy lasers and light sources, relevant to understanding brown dwarf and white dwarf interiors and inertial confinement fusion science.
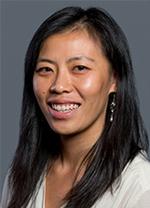
Tammy Ma
“I’m grateful to LLNL for all the opportunities, support and great working relationships. I’m honored to receive this alongside Dr. Doeppner and Dr. Xu."
Tammy Ma, a physicist in the High Energy Density Division of the NIF&PS Directorate, was selected for “outstanding scientific contributions and leadership in the field of intense laser-matter interactions and inertial fusion energy science.” At the Advanced Photon Technologies (APT) Program within NIF&PS, her team is working on high-intensity short-pulse laser-based research, with a focus on establishing new applications of these tools to support future National Nuclear Security Administration and Department of Energy missions.
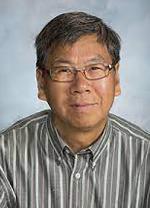
Xueqiao Xu
“It is a great honor to be recognized as a fellow of the American Physical Society. I feel very proud, and I am most grateful to those nominating me and awarding me with such distinction.”
Xueqiao Xu, a physicist in the Physics Division of the Physical and Life Sciences Directorate, was cited for “wide-ranging contributions to the understanding of the tokamak edge, including edge pedestal stability and the onset and evolution of edge localized modes and for leading the development of edge simulation models and codes.” Xu has been at the Lab for 28 years and his research focuses on theory and simulations of boundary plasma turbulence and transport for physics research of magnetic fusion energy, such as ITER (International Thermonuclear Experimental Reactor).
Long-term Impact
The LDRD program is an investment in our nation’s future, ensuring mission support that is often realized many years after an LDRD-funded project concludes. Recognizing this long-term impact of the LDRD program, we believe it is important to highlight indicators that span multiple years, demonstrating the true impact of LDRD as a national asset.
We collaborated with our colleagues from LDRD programs at other NNSA institutions to identify ways that we could best represent the long-term impact of LDRD investments. As each institution issues its LDRD program report for fiscal year 2021, we present a common set of long-term performance indicators including the content provided below.
Distinguished Member of the Technical Staff
One relevant indicator of career advancement in a science and technology field is the recognition of individuals as distinguished members of the technical staff at the institution. Individuals who receive this recognition are identified as being in the top 1% or 2% of the institution’s scientific and technical staff, similar to a lifetime achievement award, or in this case, for their contribution to the Laboratory’s mission.
At LLNL, appointment as a Distinguished Member of the Technical Staff (DMTS) is reserved for Laboratory scientists and engineers who have demonstrated a sustained history of high-level achievements in programs of importance to the Laboratory, become a recognized authority in the field, or made a fundamental and important discovery that has sustained, widespread impact.
As presented in the table on the following page, a vast majority of these distinguished staff at LLNL had early career experience with LDRD projects, which helped them develop their scientific, technical, and leadership skills.
Single-Year Statistics | Multi-Year Statistics | |||||
---|---|---|---|---|---|---|
History of DMTS Awards at LLNL | FY19 | FY20 | FY21 | FY11–15 (5 yrs) |
FY16–20 (5 yrs) |
FY11–20 (10 yrs) |
Total DMTS awards | 6 | 0 | 0 | 34 | 14 | 48 |
DMTS with LDRD roots | 6 | N/A | N/A | 27 | 14 | 41 |
% with LDRD roots | 100% | N/A | N/A | 79% | 100% | 85% |
Average years from first LDRD experience | 17.5 | N/A | N/A | 18.1 | 21.1 | 19.1 |
R&D 100 Awards
Another indicator of advancement and leadership in a scientific field is the R&D 100 Award program, which honors the top innovations of the past year. R&D 100 Awards can occur a long time after the initial ideas are developed, often during LDRD projects. Typically, it takes 5 to 10 years (or longer) from concept development to receiving an award, including the time needed to move through patenting an invention and demonstrating its commercial applications.
Single-Year Statistics | Multi-Year Statistics | |||||
---|---|---|---|---|---|---|
History of R&D 100 Awards at LLNL | FY19 | FY20 | FY21 | FY12–16 (5 yrs) |
FY17–21 (5 yrs) |
FY12–21 (10 yrs) |
Total R&D 100 Awards | 4 | 1 | 3 | 21 | 15 | 36 |
Awards with LDRD roots | 2 | 0 | 0 | 10 | 3 | 13 |
% with LDRD roots | 50% | 0% | 0% | 48% | 20% | 36% |
Average years from 1st LDRD experience | 12 | N/A | N/A | 6.9 | 11.0 | 7.9 |