Program Value
From publications to intellectual property to long-term impact, LDRD is a major contributor to the Laboratory's scientific and technological accomplishments.
- Collaborative Explorations
- Intellectual Property
- Scientific Publications
- Early Career
- Professional Fellows
- Long-Term Impact
Collaborative Explorations
External collaborations are essential to the innovative research that takes place at LLNL, including LDRD-funded projects. By collaborating with other national laboratories, academia, and industry, our investigators can engage with experts from other institutions and access world-class experimental facilities.
The following table provides our most recent data regarding formal collaborations, which we define as LDRD-funded projects where an external collaborator received LDRD funds from LLNL. In addition, our investigators frequently participate in informal collaborations with researchers at other institutions, which often involve joint scientific publications. Both types of collaborations are a key indicator of the broad intellectual engagement that is a hallmark of LLNL’s research environment.
Collaborations | FY20 | FY21 | FY22 | FY23 | FY24 |
---|---|---|---|---|---|
LDRD-funded projects with one or more formal collaborations | 78 | 88 | 79 | 81 | 79 |
Percentage of LDRD projects | 32% | 33% | 32% | 30% | 31% |
Are shapeshifting “soft machines” in our future? LLNL scientists advance light-responsive material
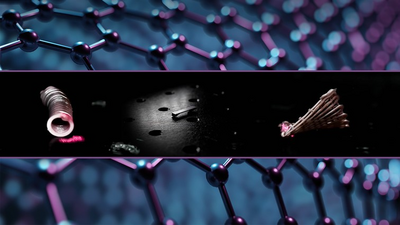
Researchers at Lawrence Livermore National Laboratory have furthered a new type of soft material that can change shape in response to light, a discovery that could advance “soft machines” for a variety of fields, from robotics to medicine.
The novel material, called a liquid crystal elastomer (LCE), is made by incorporating liquid crystals into the molecular structure of a stretchable material. Adding gold nanorods to the LCE material, scientists and engineers created photo-responsive inks and 3D printed structures that could be made to bend, crawl and move when exposed to a laser that causes localized heating in the material. The results were recently published online by Matter, and will be featured in the journal’s March 2025 print issue.
The LLNL team, along with their collaborators from Harvard University, North Carolina State University and the University of Pennsylvania, used a direct ink writing printing technique to build a variety of light-responsive objects, including cylinders that could roll, asymmetric “crawlers” that could go forward and lattice structures that oscillated. By combining shape morphing with photoresponsivity, researchers said the new type of material could change the way people think about machines and materials.
“At LLNL, we’ve focused on developing static materials and architectures for some time,” said principal investigator Caitlyn Krikorian (Cook). “We’ve made these complex types of structures like hierarchical lattices, and we’ve even started exploring more responsive materials, like shape memory polymers, that have a one-time shape memory response. But the Lab really hadn't delved deep into creating architectures that can go from a 3D-to-3D type of shape change. This project is starting to show how architecture and these novel materials can have unique modes of actuation that we haven't researched before.”
Researchers said the new material could be used to create a “soft machine” — a type of machine made from these flexible LCE composite materials — capable of responding to external stimuli and even mimicking the movements and behaviors of living organisms. Soft robots made of the shape-morphing material could crawl, swim or fly, and explore environments that are too difficult or dangerous for humans to access, like caves or outer space. Soft machines could also be used in medical applications, such as implantable devices that can adapt to the body's movements, or prosthetic limbs that move like natural limbs, and other applications that aren’t possible with machines made from rigid materials, like metal or plastic.
Researchers said the movement of the LCE material is driven primarily by a process known as photothermal actuation, which involves converting light energy into thermal energy resulting in a mechanical response from the material. Driven by the interaction between light, gold nanorods and the LCE matrix, the process enables the printed structures to exhibit dynamic and reversible movements in response to external stimuli.
“When you have this composite material — in this in case, these gold nanorods in these liquid-crystal elastomers — it has a photothermal effect,” Cook explained. “With [infrared] light, it creates a heating effect, which causes the aligned molecules to become misaligned. During that misalignment process, if there's uniform heating, you’ll have a global shape change. But in this case, we can have localized heat change, which is how you can get those localized regions of shape morphing to do things like locomotion.”
In the study, researchers used a computer vision system, involving cameras and a tracking software, to control the movement of a printed cylinder. The tracking system monitored the position of the rolling cylinder and continuously adjusted the position of the laser to raster the edge of the cylinder. This continuous tracking and adjustment allowed the cylinder to maintain its rolling motion in a controlled manner.
By leveraging computer vision with the photothermal actuation of the cylinder, the researchers achieved a sophisticated level of manipulation of the soft machine's movement, showcasing the potential for advanced control systems in the field of soft robotics and soft machines. The team also showed that responsivity could be controlled so the soft machines could perform useful tasks, such as a moving cylinder carrying a wire.
“[Lead author Ford] did some awesome work in using computer vision to control the locomotion of the printed cylinder and using a rastering laser to force it to move,” said co-author Elaine Lee. “But once you start to get into much more complex motion — like using various rastering speeds and light intensities on a printed lattice, causing it to move in various different modes — those were actually outside of what our high performance computing (HPC) simulations were able to predict, because those codes are expecting a uniform heating or stimuli on that lattice. So, using computer vision and machine learning to learn the actuation speeds, and what doses of light can cause locomotion from that printed architecture, will push us a lot further in understanding how our materials will respond.”
Researchers said there are still some challenges that need to be overcome before the material can be used in practical applications. The team found that structures they created could flip over or exhibit other unpredictable motion, thereby making it difficult to design specific modes of motions. They said they will continue to work on models that can describe the complex motion to better design future machines and develop new materials and manufacturing techniques to create soft machines that are more durable, reliable and efficient for a variety of applications. New control systems and computer algorithms also could enable soft machines to move and interact with their environment in a more intelligent and autonomous way, they said.
Cook said the team is looking at incorporating responses to different types of stimuli, beyond thermal and light stimuli, into areas like humidity and energy absorption, and conditions that the material might experience in space. She added that the team is looking at starting a new Strategic Initiative at the Lab to focus on autonomous materials and “move the needle” towards sentient materials.
“We’re all thinking about ways to make materials more autonomous; sentient materials that can sense, respond, be programmed, learn, decide and communicate,” Cook said. “These liquid crystal elastomers are responsive materials — they’re able to sense a stimuli and respond and will respond repeatedly every time — but it doesn't have a sense of memory or a way to learn the repeated stimuli and respond accordingly. It doesn't have a means to communicate yet, other than potentially being able to pair it with some type of mechanical computing. These are really the materials that we're striving towards, and this might be a five- to 10-year timespan of effort.”
LDRD Project Title: Shape Changing of Responsive Elastomer Structures
Principal Investigator:
Caitlyn Krikorian Cook
LDRD Project:
22-ERD-030
Intellectual Property
Year after year, projects sponsored by LDRD achieve a disproportionately large percentage of the patents and copyrights issued for LLNL research. As illustrated in the following tables, LDRD-funded work has been key in developing more than half of the Laboratory’s patents, one-third of the Laboratory’s copyrights (chiefly computer code), and more than half of the Laboratory’s records of invention.
Patents | FY20 | FY21 | FY22 | FY23 | FY24 |
---|---|---|---|---|---|
All LLNL patents | 200 | 166 | 166 | 197 | 200 |
LDRD patents | 131 | 96 | 103 | 116 | 126 |
LDRD patents as a percentage of total | 66% | 58% | 62% | 58% | 63% |
Copyrights | FY20 | FY21 | FY22 | FY23 | FY24 |
---|---|---|---|---|---|
All LLNL copyrights | 138 | 125 | 142 | 166 | 201 |
LDRD copyrights | 31 | 42 | 47 | 44 | 66 |
LDRD copyrights as a percentage of total | 22% | 34% | 33% | 26% | 33% |
Records of Invention | FY20 | FY21 | FY22 | FY23 | FY24 |
---|---|---|---|---|---|
All LLNL records | 126 | 89 | 70 | 120 | 119 |
LDRD records | 56 | 53 | 40 | 64 | 61 |
LDRD records as a percentage of total | 44% | 60% | 57% | 53% | 51% |
Machine learning optimizes high-power laser experiments
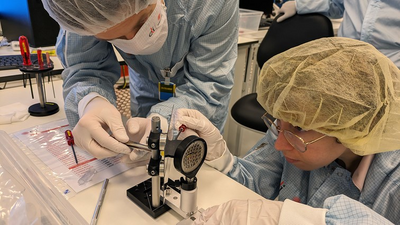
Commercial fusion energy plants and advanced compact radiation sources may rely on high-intensity high-repetition rate lasers, capable of firing multiple times per second, but humans could be a limiting factor in reacting to changes at these shot rates.
Applying advanced computing to this problem, a team of international scientists from Lawrence Livermore National Laboratory (LLNL), Fraunhofer Institute for Laser Technology (ILT) and the Extreme Light Infrastructure (ELI ERIC) collaborated on an experiment to optimize a high-intensity, high-repetition-rate laser using machine learning.
"Our goal was to demonstrate robust diagnosis of laser-accelerated ions and electrons from solid targets at a high intensity and repetition rate," said LLNL’s Matthew Hill, the lead researcher. "Supported by rapid feedback from a machine-learning optimization algorithm to the laser front end, it was possible to maximize the total ion yield of the system."
The researchers trained a closed-loop machine learning code developed by LLNL’s Cognitive Simulation team on laser-target interaction data to optimize the laser pulse shape, allowing it to make adjustments as the experiment ran. Data generated during the experiment was fed back into the machine learning-based optimizer, allowing it to tweak the pulse shape on the fly.
The laser fired every 5 seconds, consistently exceeding laser intensities of 3x1021 W/cm2 its focus, stopping after 120 shots when the copper target foil had to be replaced. During this time, the researchers also inspected the diagnostics for damage and assessed debris accumulation from vaporized targets. The team conducted experiments at ELI for three weeks, with experimental runs lasting approximately 12 hours per day, during which the laser would fire up to 500 shots.
The experiment took place at the ELI Beamlines Facility in the Czech Republic, where the researchers utilized the state-of-the-art High-Repetition-Rate Advanced Petawatt Laser System (L3-HAPLS) to generate protons in the ELIMAIA laser-plasma ion accelerator. Focusing on the goal of applying machine learning to a high-rate-laser experiment, the team simplified aspects of the experiment as much as possible, like using a robust, simple copper foil target.
"By harnessing the HAPLS and pioneering machine learning techniques, we embarked on a remarkable endeavor to further comprehend the intricate physics of laser-plasma interactions,” said Constantin Haefner, managing director of Fraunhofer ILT.
More than 4,000 shots were fired during the campaign, allowing statistical analysis to be performed on the results and demonstrating optimization of ion yield above the already-impressive nominal baseline performance.
Using machine learning was a new experience for the experimental physicists. “It becomes a spectator sport,” Hill said. “We watched the data coming in and tried to guess what the optimizer would do. It’s very different than an experiment with manual intervention.”
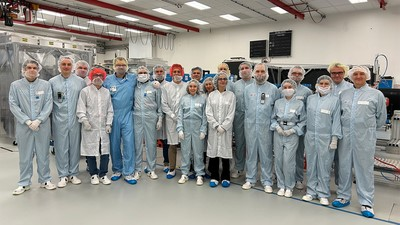
LLNL becomes a L3-HAPLS user
The L3-HAPLS laser has excellent laser performance repeatability, displaying exceptionally stable alignment, focal spot quality and the ability to generate intense laser pulses at a high repetition rate to drive the generation of secondary sources such as electrons, ions and x-rays.
"The successful execution of such a complex experiment showcases the cutting-edge quality and reliability of the L3-HAPLS laser system,” said Bedrich Rus, chief laser scientist at ELI Beamlines.
LLNL developed the HAPLS laser as part of a bilateral agreement with ELI Beamlines, with first light from the system after delivery and installation in the Czech Republic in 2017. This was only the second user experiment at the facility, having been awarded time through a competitive worldwide call for proposals, now issued twice annually and attracting hundreds of applications.
Lengthy preparation pays off
In addition to Hill, the LLNL team of Elizabeth Grace, Franziska Treffert, James McLoughlin, Isabella Pagano, Abhik Sarkar, Raspberry Simpson, Blagoje Djordjevic, Matthew Selwood, Derek Mariscal, Jackson Williams and Tammy Ma spent about a year preparing for the experiment with the Fraunhofer ILT and ELI Beamlines teams. In addition to local facility diagnostics, the Livermore team fielded several instruments developed under the Laboratory Directed Research and Development Program, including the REPPS magnetic spectrometer, PROBIES ion beam imaging spectrometer, a rep-rated scintillator imaging system and rep-rated X-ray spectrometer.
To follow export control regulations, the machine learning code had to be run at LLNL to segregate it from computer systems at ELI Beamlines. This created a challenge of rapidly transferring data between the Czech Republic to LLNL to keep pace with the laser.
Sarkar helped engineer the trans-Atlantic coupling between computers at LLNL and at ELI for real-time closed-loop operation. “Thanks to hard work on both sides, we came up with a solution,” he said. “On the computing side, this opens up new possibilities for remote operation.”
The experiment was very successful, generating robust data from the first day. In future experiments, the team hopes to allow machine learning to control additional parameters like the focal spot and to use the approach at different experimental facilities.
More broadly, the success of coupling state-of-the-art laser technology with machine learning techniques could open new avenues for advancements in various fields such as fusion energy, medical therapy, materials science and non-destructive analysis in the field of cultural heritage and archaeology.
LDRD Project Titles: Autonomous Laser Optimization for High-Repetition-Rate Applications
Principal Investigator:
Matthew Hill
LDRD Project:
24-ERD-041
Scientific Publications
Laboratory scientists and engineers publish more than a thousand papers each year in a wide range of peer-reviewed journals, of which LDRD-funded work accounts for a large portion. The numerous publications made possible through LDRD-sponsored research help the Laboratory maintain a strong presence in the broader scientific community, extending the impact of LDRD research beyond the DOE mission space into the wider scientific arena. In addition, the impact of these publications documenting LDRD project results extends long after articles appear in the journals, increasing the value of LDRD investments in these projects.
Journal Articles | FY20 | FY21 | FY22 | FY23 | FY24 |
---|---|---|---|---|---|
All LLNL articles | 1,149 | 1,256 | 1,149 | 1,218 | 1,004 |
LDRD articles | 428 | 509 | 490 | 495 | 409 |
LDRD articles as a percentage of total | 37% | 41% | 43% | 41% | 41% |
Modeling CO2 electrolysis
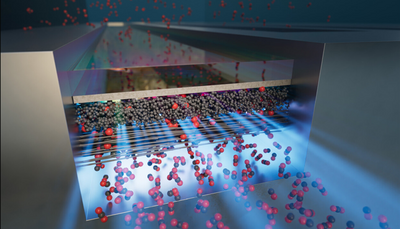
LLNL researchers and collaborators are working under a Laboratory Directed Research and Development-funded initiative (22-SI-006) to design next-generation electrochemical reactors for industrial CO2 conversion using computationally driven algorithms.
These electrolyzers can produce mixtures of different carbon-based products depending on the catalyst used. Copper catalysts, for example, can produce ethylene — the most common carbon-based commodity chemical in the world. It is the key component in a myriad of household and construction products from water bottles, carpeting, and toys to pipes, insulation, and many more.
Silver catalysts, on the other hand, can produce carbon monoxide and hydrogen, a mixture known as syngas, which is commonly used to make fuels and chemicals. In one study, published on the cover of ChemElectroChem, LLNL researchers developed Multiphysics models for two different designs — a zero-gap membrane electrode assembly (MEA) and a planar electrode with silver catalysts.
Historically, MEA electrolyzers (the device that is used to electrically transform the CO2 to produce products like ethylene and syngas) have offered significantly improved performance due to their "zero-gap" design. However, they have also been known to accumulate liquid in porous regions of the cell, a phenomenon known as flooding, which slows down the reaction rate and decreases the efficiency of the electrolyzer.
The team’s research is helping to evaluate the pros and cons of these different electrochemical conversion solutions, helping them to understand the factors associated with these limitations as they work to build an end-to-end model that simulates CO2 mass transfer across an industrialized reactor from start to finish. With this model, the researchers can optimize the reactor by simulating how different “ingredients” (catalyst used, device design, etc.) affect the CO2 conversion process.
LDRD Project Title: The Science of Scale-Up: Accelerated Scaling of Materials and Manufacturing Solutions
Principal Investigator:
Sarah Baker
LDRD Project:
22-SI-006
Early Career Opportunities: Students and Postdoctoral Fellows
By funding exciting, potentially high-payoff projects at the frontiers of science, the LDRD Program attracts top talent in new and emerging fields of science and technology. As shown in the following tables, LDRD investments contribute to the health and robustness of LLNL’s student and postdoctoral researcher programs.
Students | FY20 | FY21 | FY22 | FY23 | FY24 |
---|---|---|---|---|---|
Students supported by LDRD | 101 | 136 | 149 | 185 | 216 |
Percentage of all students | 18% | 24% | 23% | 24% | 26% |
Postdoctoral Researchers | FY20 | FY21 | FY22 | FY23 | FY24 |
---|---|---|---|---|---|
Postdoctoral researchers supported by LDRD ≥10% of their time | 208 | 208 | 240 | 213 | 234 |
Percentage of all postdoctoral researchers | 63% | 54% | 56% | 51% | 58% |
LDRD postdoctoral researchers converted to full staff | 60 | 50 | 72 | 56 | 44 |
Percentage of all conversions | 77% | 82% | 77% | 70% | 66% |
3D-printed electrode is all charged up
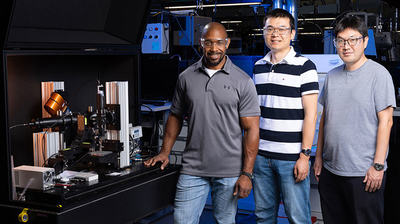
The architectural design of electrodes offers new opportunities for next-generation electrochemical energy storage devices (EESDs) by increasing surface area, thickness and storage capacity.
But conventional thick electrodes increase ion diffusion length and cause larger ion-concentration gradients, limiting reaction kinetics, including storage capacity.
To overcome these challenges, Lawrence Livermore National Laboratory (LLNL) scientists and collaborators at the University of California, Santa Cruz 3D-printed a new and compact device configuration with two interpenetrated, individually addressable electrodes, allowing precise control over the geometric features and interactions between the electrodes.
Using powerful high-performance computing facilities at LLNL, the team demonstrated through computational simulation that the interpenetrated electrode design improves ion-diffusion kinetics in EESDs by shortening the ion-diffusion length and reducing ion-concentration inhomogeneity.
“The device with interpenetrated electrodes outperformed the traditional separate electrode configuration, enhancing both volumetric energy density and capacity retention rate,” said LLNL postdoc Longsheng Feng, co-author of a paper appearing in Nano-Micro Letters.
This free-standing device structure also avoids short-circuiting without needing a separator. The feature size and number of interpenetrated units can be adjusted during printing to balance surface area and ion diffusion.
The team used a zinc manganese dioxide battery as a model system and found that the device outperforms conventional separate electrode configurations, improving volumetric energy density by 221% and exhibiting a higher capacity retention rate of 49% compared to 35% at temperatures from 20 to 0 °C.
“Our study introduces a new EESD architecture applicable to lithium-ion, sodium-ion batteries, supercapacitors and other storage systems,” Feng said. “To our knowledge, this is the first time that interpenetrated lattices have been used for architectural EESDs.”
Significantly ordered, periodically entangled two electrodes occupying the same free volume are close to each other throughout the whole structure regardless of its thickness. This structural characteristic is anticipated to enhance the ion diffusion kinetics during the charging and discharging processes.
“This new tool kit of materials and designs will enable high-performance energy storage in conditions relevant to national security missions and the broader energy storage community,” said LLNL scientist Marcus Worsley, a co-lead author.
Other LLNL authors include Tae Wook Hoo and Cheng Zhu.
LDRD Project Title: Advanced Energy Storage Technologies for Extreme Conditions
Principal Investigator:
Marcus Worsley
LDRD Project:
23-SI-002
Professional Fellows
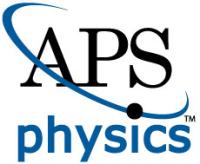
One relevant indicator of advancement and leadership in a scientific field is the election of individuals as fellows of professional societies. This indicator reflects success for both the individual researcher and the Laboratory as a whole.
American Physical Society (APS) fellowships are awarded based on scientific merit and impact over an extended period, and the evaluation process relies on nomination and recommendation by peers. As such, data regarding the history of APS fellowships awarded to LLNL physicists provide an important indicator regarding the key role that the LDRD Program plays in developing the technical, scientific, and leadership skills of early career staff. As presented in the following table, for fiscal year 2023, 100% of the new APS Fellows from LLNL have early career LDRD experience.
Because the quantity of awards each year is a small number, we also present multi-year statistics. For example, over the last 20 years, more than 90% of the APS Fellows at LLNL had early career LDRD experience.
Single-Year Statistics | Multi-Year Statistics | |||||
---|---|---|---|---|---|---|
History of APS Fellows at LLNL | FY22 | FY23 | FY24 | FY15–19 (5 yrs) |
FY20–24 (5 yrs) |
FY15–24 (10 yrs) |
Total APS awards | 2 | 2 | 3 | 24 | 14 | 38 |
Awards with LDRD roots | 2 | 2 | 3 | 23 | 14 | 37 |
% with LDRD roots | 100% | 100% | 100% | 96% | 100% | 97% |
Average years from first LDRD experience | 15 | 15.5 | 8.3 | 15.6 | 13 | 14.6 |

Tracing impact to LDRD roots
Throughout this section, we mention “LDRD roots.” Much discussion with principal investigators has transpired about what it means for an accomplishment to have LDRD roots. A simple case would be if an idea for an invention arises during an LDRD project and work on the invention is completed during the period of LDRD investment. But R&D often does not advance on such a short timescale. In general, an accomplishment (invention, paper, capability, etc.) is determined to have LDRD roots if at least one LDRD project needed to occur for the accomplishment to take place. In other words, if one can identify an LDRD project that was critical to the accomplishment, then it is considered to have LDRD roots.
2024 APS Fellows at LLNL
Three Lab scientists named 2024 APS Fellows
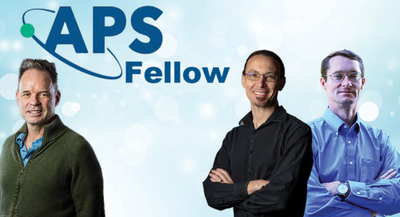
Lawrence Livermore National Laboratory (LLNL) scientists Daniel Casey, Daniel Clark and Raymond Smith have been named 2024 American Physical Society (APS) Fellows.
Casey was selected for “outstanding contributions to the understanding of the stagnation conditions required to achieve ignition.” Clark was honored for “extensive contributions to inertial confinement fusion state-of-the-art implosion modeling, innovative ignition designs, novel applications of laser-plasma interactions, and the scientific understanding of hydrodynamic instabilities.” Smith was selected for “for pioneering dynamic ramp-compression experiments on high-energy laser facilities, resulting in significant discoveries in high-pressure materials physics and planetary science.”
The APS Fellowship Program was created to recognize members who may have made advances in physics through original research and publication or made significant innovative contributions in the application of physics to science and technology. They also may have made significant contributions to the teaching of physics or service and participation in the activities of the society.
Fellowship is a distinct honor signifying recognition by one's professional peers. Each year, no more than one half of one percent of the society’s membership (excluding student members) is recognized by their peers for election to the status of fellow of the American Physical Society.
DANIEL CASEY
Experimental excellence in fusion research
“I am humbled and incredibly honored to be named an APS Fellow,” he said. “It is often said that scientists stand on the shoulders of giants. Indeed, I am indebted to my friends and colleagues who have helped me at every stage of my research and am deeply grateful.”
Daniel Casey is a physicist who works in the National Ignition Facility (NIF) and Photon Science Directorate and is the low mode working group lead for the Inertial Confinement Fusion (ICF) program. He was recently appointed group leader for the Implosions & Stagnations (Hydrodynamics) group. His work focuses on diagnosing and assessing the impact of asymmetries in ICF implosions.
DANIEL CLARK
Leading the charge in ICF design and modeling
“To join so many of my exceptional colleagues in the ICF program in receiving the APS Fellowship is a profound honor,” Clark said. “The recognition not only acknowledges my individual contributions to the field but also highlights the Laboratory’s collaborative efforts. This honor inspires me to continue our pursuit of groundbreaking advancements in plasma physics."
Daniel Clark is a physicist who works in the Strategic Deterrence Directorate at LLNL and specializes in ICF design and modeling. He has served as the design lead for several experimental campaigns at NIF and, from 2013 to 2021, led the capsule modeling working group within the ICF program.
RAYMOND SMITH
Pioneering dynamic ramp-compression techniques
“I’m honored to be selected as an APS Fellow, and I deeply appreciate this recognition from the American Physical Society. This distinction reflects the collaborative efforts of my colleagues, mentors and students who have contributed to my research journey. It motivates me to continue pushing the boundaries of scientific discovery and to foster a spirit of curiosity and innovation within the broader physics community,” Smith said."
Raymond Smith is a physicist who works in the Physical and Life Sciences Directorate and is the lead principal investigator for the NIF TARDIS X-ray diffraction platform, which has provided direct measurements of crystal structure and microstructural texture at terapascal (TPa) pressures (1 TPa is equivalent to 10 million Earth atmospheres).
Long-Term Impact
The LDRD Program is an investment in our nation’s future, ensuring mission support that is often realized many years after an LDRD-funded project concludes. Recognizing this long-term impact of the LDRD Program, we believe it is important to highlight indicators that span multiple years, demonstrating the true impact of LDRD as a national asset.
We collaborated with our colleagues from LDRD Programs at other NNSA institutions to identify ways that we could best represent the long- term impact of LDRD investments. As each institution issues its LDRD Program report for fiscal year 2024, we present a common set of long-term performance indicators including the content provided below.
Distinguished Member of the Technical Staff
One relevant indicator of career advancement in a science and technology field is the recognition of individuals as distinguished members of the technical staff at the institution. Individuals who receive this recognition are identified as being in the top 1% or 2% of the institution’s scientific and technical staff, similar to a lifetime achievement award, or in this case, for their contribution to the Laboratory’s mission.
At LLNL, appointment as a Distinguished Member of the Technical Staff (DMTS) is reserved for Laboratory scientists and engineers who have demonstrated a sustained history of high-level achievements in programs of importance to the Laboratory, become a recognized authority in the field, or made a fundamental and important discovery that has sustained, widespread impact.
As presented in the following table, a vast majority of these distinguished staff at LLNL had early career experience with LDRD projects, which helped them develop their scientific, technical, and leadership skills.
Single-Year Statistics | Multi-Year Statistics | |||||
---|---|---|---|---|---|---|
History of DMTS Awards at LLNL | FY22 | FY23 | FY24 | FY15–19 (5 yrs) |
FY20–24 (5 yrs) |
FY15–24 (10 yrs) |
Total DMTS awards | 26 | 0 | 23 | 25 | 49 | 74 |
DMTS with LDRD roots | 20 | N/A | 12 | 23 | 32 | 55 |
% with LDRD roots | 77% | N/A | 52% | 92% | 65% | 74% |
Average years from first LDRD experience | 16.4 | N/A | 14.5 | 20.5 | 15.7 | 17.7 |
R&D 100 Awards
Another indicator of advancement and leadership in a scientific field is the R&D 100 Award program, which honors the top innovations of the past year. R&D 100 Awards can occur a long time after the initial ideas are developed, often during LDRD projects. Typically, it takes 5 to 10 years (or longer) from concept development to receiving an award, including the time needed to move through patenting an invention and demonstrating its commercial applications.
The LDRD Program is an investment in our nation’s future, ensuring mission support that is often realized many years after an LDRD-funded project concludes. Recognizing the is long-term impact of the LDRD Program, we believe it is important to highlight indicators that span multiple years, demonstrating the true impact of LDRD as a national asset.
Single-Year Statistics | Multi-Year Statistics | |||||
---|---|---|---|---|---|---|
History of R&D 100 Awards at LLNL | FY22 | FY23 | FY24 | FY15–19 (5 yrs) |
FY20–24 (5 yrs) |
FY15–24 (10 yrs) |
Total R&D 100 Awards | 3 | 3 | 3 | 17 | 13 | 30 |
Awards with LDRD roots | 2 | 3 | 2 | 6 | 7 | 13 |
% with LDRD roots | 67% | 100% | 67% | 35% | 54% | 43% |
Average years from 1st LDRD experience | 7.5 | 14.7 | 13.5 | 7.3 | 12.3 | 10 |
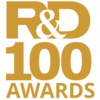
The renowned R&D 100 competition, now in its 62nd year, received entries from 16 countries and regions around the world.
Lab scientists win three R&D 100 awards
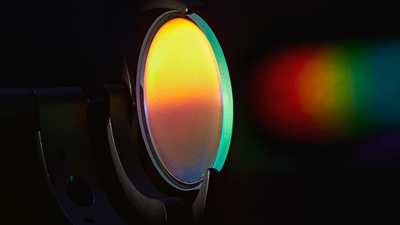
Lawrence Livermore National Laboratory (LLNL) scientists and engineers have earned three awards among the top 100 inventions worldwide.
The trade journal R&D World Magazine recently announced the winners of the awards, often called the “Oscars of innovation,” recognizing new commercial products, technologies and materials that are available for sale or license for their technological significance.
With this year’s results, the Laboratory has now collected a total of 182 R&D 100 awards since 1978. Submitted through LLNL’s Innovation and Partnerships Office, these awards recognize the impact that Livermore innovation, in collaboration with industry partners, can have on the U.S. economy as well as globally.
This year’s LLNL R&D 100 awards include a spectral beam combining optic that enables a single, high-power beam with unparalleled compactness and damage resistance; an open-source memory-mapping library with increased power and flexibility; and a user-level file system for high-performance computing systems.
“The R&D 100 awards highlight the most innovative, game-changing technologies, and it is wonderful to see LLNL teams being recognized,” Lab Director Kim Budil said. “Having three projects selected for this honor shows clearly the high degree of excellence and ingenuity our scientists and engineers bring to creating impactful solutions to important challenges.”
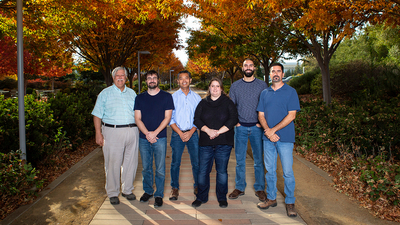
Optics breakthrough improves laser performance
Demand for high-power laser sources with diffraction-limited beam quality is increasing as material processing techniques such as marking, cutting, welding and drilling often require a laser beam that can transmit over distance while maintaining excellent quality, to minimize undesired beam spreading.
The increased demand is leading to a significant scaling effort for laser systems’ output power to reach hundreds of kilowatts and even megawatts. However, challenges to scaling the output power include removing heat waste, maintaining beam quality and avoiding damage to output optics.
The Extreme-power, Ultra-low-loss, Dispersive Element (EXUDE) Elite optical element addresses these challenges by concentrating light from multiple lasers with different wavelengths into a single, high-power beam with unparalleled compactness and damage-resistance.
EXUDE Elite significantly improves upon the original EXUDE technology (winner of a 2014 R&D 100 award), which made it possible to use Spectral Beam Combining (SBC) for near-diffraction-limited quality laser systems with first-ever output powers approaching megawatt levels. EXUDE Elite combines fiber laser beams via transmission through a fused silica optic, which is achieved in a less-expensive, more compact system with a 100-fold improvement to the damage threshold.
EXUDE Elite is a breakthrough that suggests a paradigm shift in high-power laser technology. The lower price and improved overall optics performance of EXUDE Elite offer the opportunity for wider access to SBC for industrial laser systems, ushering the laser power-scaling effort forward on a larger scale than ever before.
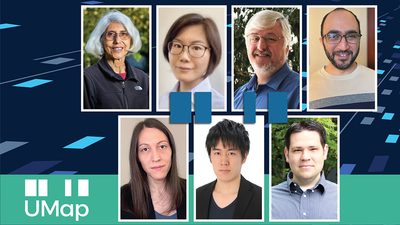
High-performance memory mapping library
Supercomputing and high performance computing (HPC) are critical for enabling and accelerating scientific research. Accompanying the exponential growth in compute power is a complex and deep memory hierarchy, which creates inefficiencies when moving data from its storage location to the processor. Because of this, supercomputer applications face large, complex data problems from both the computer system (complex hierarchy, memory, and storage) and the application workload.
To address this, a team of LLNL computer scientists developed the UMap user-level library as part of the U.S. Department of Energy’s Exascale Computing Project, specifically the Argo Project, in which UMap is a key solution for deep and complex memory and storage systems.
UMap offers a high-performance, application-configurable, unified memory-like interface to diverse datastores located across memory-storage hierarchical levels or even across a network. It is purpose-built for high performance through a highly optimized and configurable design and is recognized as a leading solution for memory mapping diverse and large datastores.
Freely available as open source, UMap is significantly aiding the scientific community and has been adopted in many high-impact scientific applications in industry, academia and national labs.
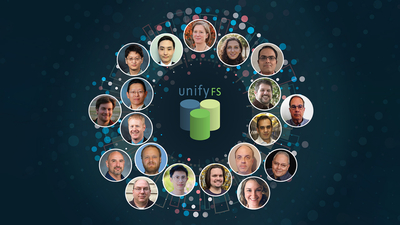
Speeding simulation modeling for impact
UnifyFS, another R&D 100 award winner, was developed by a team from LLNL, Oak Ridge National Laboratory and the University of Illinois’ National Center for Supercomputing Applications.
When HPC applications are used to simulate real-world phenomena, the results from predictive models can be used by policy makers to inform important, life-saving decisions. However, scientific simulations can take hours, days or even weeks to compute due to slowdowns in the dynamic input/output (I/O) communication between the supercomputer and the file.
The UnifyFS file system enables HPC science applications to perform I/O operations many times faster than they could with traditional methods.
UnifyFS is a temporary file system that uses fast storage tiers on supercomputers to quickly store and access application data, so that applications can produce their results in less time. In addition to providing high performance I/O operations, UnifyFS is easy to use and teams can leverage it without an administrator’s help or changing their code to accommodate different types of HPC systems. Also available as open source and production-ready, UnifyFS makes I/O fast and easy so users can focus on their science.
UnifyFS collaborators are Lawrence Livermore and Oak Ridge National Laboratories, as well as the National Center for Supercomputing Applications at the University of Illinois.
LDRD’s Long-Term Program Impact
As part of the Lawrence Livermore National Laboratory LDRD Annual Report, each year we include a chart showcasing the long-term impact of LDRD on the LLNL Missions and Science and Technology capabilities. This year we highlight LLNL’s activities to innovate the next generation laser science at LLNL. The lab has been a leader in laser materials and laser science since the laboratory was formed; over the last 10 years, seeded by the LDRD Program, LLNL has created a new laser research and development ecosystem for high pulse energy short wave infrared (SWIR) lasers at ~2μm wavelength and their applications in national security and beyond. Focusing on advances in optical materials, laser diode pumping, and laser system design, LLNL has pushed the frontiers of laser technology in the ~2μm wavelength region to obtain a world record pulse energy (>100J) and world record peak powers (>1TW). These new capabilities are being studied and exploited for NNSA and National Security applications in radiography and for deeper understanding of important physics phenomena such as laser-material interactions. It also drives new research and development opportunities in extreme ultraviolet lithography (EUVL) as well as imaging and detection of nuclear materials and deeply buried geophysical structures.
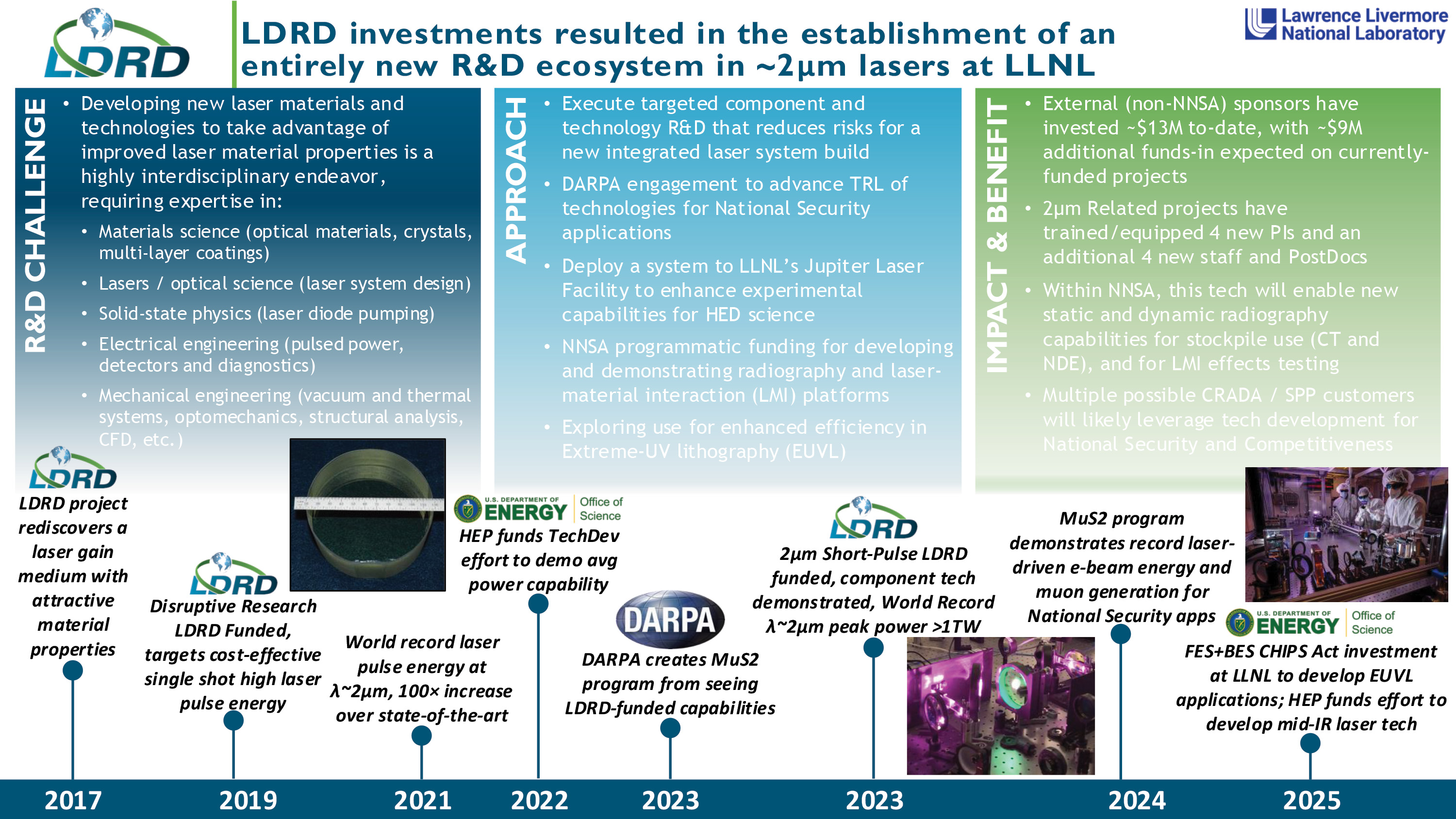