Program Accomplishments
LDRD-funded research explores the frontiers of science and technology in emerging mission spaces, with projects guided by an extremely creative, talented team of scientists and engineers.
Featured Research
LDRD funded 244 projects in fiscal year 2020. Brief summaries of each project can be found in the Project Highlights section of our report. Here, we provide a closer look at a handful of projects that underscore the exciting, innovative research in this year’s LDRD portfolio.
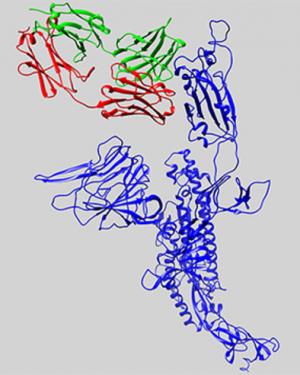
Livermore’s LDRD program invests in research aimed at meeting some of the nation’s most difficult challenges. Shortly after the COVID-19 pandemic emerged in early 2020, these internally directed funds enabled the Laboratory to rapidly launch new projects and redirect the focus of existing projects to help the nation respond to the pandemic.
According to Doug Rotman, who leads the LDRD program at Livermore, “We had several scientists contact us with ideas regarding how they could help our Laboratory respond to the national COVID challenge, including emerging needs related to therapeutic targets and designs. Working closely with science leaders across LLNL, the LDRD program rigorously and quickly evaluated proposals and approved funding needed to start work.”
The LDRD-sponsored projects leveraged LLNL’s capabilities in high-performance computing, simulation, and data science—combined with Livermore’s expertise in bioscience and bioengineering—to identify risk factors for COVID-19 patients and accelerate development of medical countermeasures.
LLNL combines bioinformatics expertise and supercomputing resources to explore antiviral drug design
Soon after the pandemic was recognized as a global crisis, leaders of three LDRD-funded projects quickly expanded the scope of their projects, focusing on efforts to identify new antibodies and antivirals that could effectively treat a COVID-19 infection.
Armed with the predicted protein structure of especially important parts of the viral replication machinery and a handful of antibodies known to bind and neutralize SARS-CoV-1 (a similar coronavirus that causes Severe Acute Respiratory Syndrome), researchers used LLNL’s high-performance computing platforms and artificial intelligence resources to virtually screen antibodies capable of binding to SARS-CoV-2 virus receptors and neutralizing them. Their novel approach integrated bioinformatic modeling and molecular simulations, driven by a machine-learning algorithm, to identify antibody candidates.
These techniques provided the team with the speed and scalability needed to search and evaluate huge numbers of possible antibody designs and reduce the possibilities to 20 initial sequences predicted to target SARS-CoV-2. This approach sped up the process considerably, and enabled scientists to focus their search on areas they might otherwise have overlooked—in the appropriate design space.
“Being able to rapidly estimate antibody structures makes it possible to follow up on promising predictions as they emerge,” said LLNL data scientist Tom Desautels, principal investigator for one of the LDRD-funded projects. “Searching a design space of this size just wouldn’t be feasible without powerful computing resources because the volume of decisions is too high.”
Experimental validations of top candidates improve countermeasure development
The virtual screening of millions of antibody and antiviral candidates made it possible for the team to identify an initial set of promising compounds in just a few weeks. The next step involved a team of LLNL experts in virology, immunology, and molecular biology, who synthesized and validated the efficacy of the top candidates through experiments—another example of work that was quickly launched by expanding the scope of an existing LDRD-funded project.
Using binding assays and biolayer interferometry technology, the experimental team conducted precise measurements to test how well the molecules bind to their target. They used these measurements to rank therapeutic candidates and further narrow the pool of computationally identified candidates with the antiviral properties needed to fight this disease.
The experimental team tailored their research to ongoing computational efforts, so that the two aspects of the research worked synergistically, with validated results feeding back into the predictions.
Multimodal machine-learning tools predict risk factors for COVID-19 patients
Investigators of another LDRD-funded project also shifted their focus after the pandemic began. A team of computational scientists was studying ways to use multimodal machine learning to fuse information from disparate sources and learn the underlying temporal structure of the data. They had already been exploring ways to apply machine-learning tools to existing medical datasets in order to more effectively predict clinical outcomes.
Lead investigator Priyadip Ray realized that his team could adapt this work to conduct predictive risk analyses for COVID-19 patients. Using the dynamic modeling framework they had started developing, the team fused genomic data regarding virus mutations with medical datasets regarding microbes present in clinical samples obtained from COVID-19 patients.
As their work continues, they are collaborating with data science experts at LLNL to create a diagnostic-testing knowledge base that the research community can use to predict the variables associated with COVID-19 disease progression. The data repository will help medical providers detect unforeseen, potentially actionable changes to a patient’s health and identify optimal treatment options.
Diagnostic tool adapted to screen for COVID-19 co-infections
LDRD-funded research often focuses on innovative solutions with a broad range of potential applications. One example of this ability to tailor technology initially developed with LDRD funds involves a diagnostic tool known as the Lawrence Livermore microbial-detection array (LLMDA), developed in 2008. This high-throughput, nucleic-acid detection technology can simultaneously analyze and identify up to 12,000 microbial species in a single test.
LLNL scientists adapted LLMDA to analyze nucleic acids extracted from COVID-19 oral and nasal samples and identify the presence of other pathogens. Their work involved designing a unique COVID-19 signature that could be incorporated into the existing LLMDA technology to detect the presence of the SARS-CoV-2 virus. They validated the new capability using LLMDA to analyze samples that had already tested positive for COVID-19 using more traditional diagnostic methods, and then looked for the presence of other pathogens in the samples. They were able to identify several types of co-infections, such as Influenza A and Streptococcus pneumoniae.
The research team anticipates that collecting this co-infection data can help scientists better understand how the presence of other pathogens may affect a person’s susceptibility to COVID-19 infection, as well as how they respond when infected with the SARS-CoV-2 virus.
Related LDRD Projects
- Identifying Potential Antiviral Small Molecules Against the Coronavirus Disease 2019 (PI Felice Lightstone)
- Active Learning for Rapid Design of Vaccines and Antibodies (PI Tom Desautels)
- Rapid Computational Identification of Therapeutic Targets for Pathogens (PI Jonathan Allen)
- Building a Computational and Experimental Rapid Response Pipeline to Counter the Coronavirus Disease 2019 Outbreak and Emerging Biothreats (PI: Brent Segelke)
- A Deep Bayesian Active Learning Framework for Temporal Multimodal Data (PI Priyadip Ray)
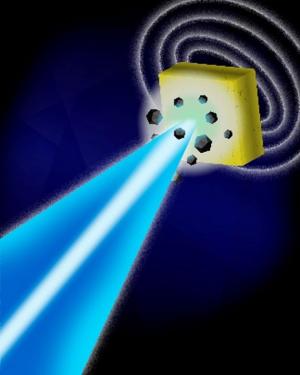
Unlocking the mysteries of high-explosive chemistry may hold the key to addressing a range of mission-relevant challenges, including nonproliferation and counterterrorism. During an LDRD-funded research project that recently concluded, LLNL scientists moved beyond computational predictions regarding the behavior of high explosives. They obtained direct measurements of chemical reactions involving an insensitive high explosive—data that is unprecedented in high explosive (HE) science.
The research team conducted the first-ever experiment of this kind, using the world’s most energetic laser to study a high-explosive sample. During their experiments at LLNL’s National Ignition Facility (NIF), investigators captured novel diagnostic data that will help scientists better understand and predict HE behavior.
According to Lara Leininger, director of LLNL’s Energetic Materials Center (EMC) and principal investigator for this LDRD project, “The laser shot is the first in a series of experiments that will transform the Lab’s understanding of high explosives by producing never-before-captured experimental data quantifying the response of laser-driven high explosives during reaction.” They captured detailed, temporally and spatially resolved data regarding the evolving chemistry of a reacting insensitive high explosive.
These expanded diagnostic capabilities will allow LLNL to investigate the structure of HE detonation products during the detonation, while also helping to validate predictive models that play a key role in understanding HE detonation and safety.
During the first phase of the three-year project, which started in 2017, the multidisciplinary team developed novel diagnostic techniques to measure in-situ, dynamic, laser-driven, high-explosive reactions. Their first experiment took place at LLNL’s Jupiter Laser Facility.
According to LLNL physicist Jon Eggert, who led the project’s high-energy-density experiments, the team was able to adapt an existing diagnostic platform, known as TARDIS (TARget Diffraction In Situ), to conduct the experiments. “It was gratifying to see that a platform we developed for very different scientific and programmatic applications, including its dual-probe and large spot-size options, could be used in this new research space,” said Eggert.
In the second phase, they evaluated concepts during a series of shots at the Omega Laser Facility at the University of Rochester. They also investigated target preparation, configuration, and diagnostic setups.
The capstone phase of the project was performed at NIF, integrating the techniques developed in the first two phases with NIF’s advanced diagnostic systems, which enabled the team to extract the required experimental data.
The sample used in the NIF shot consisted of a non-detonable quantity of less than 7 milligrams of single-crystal TATB (1,3,5-triamino-2,4,6-trinitrobenzene), a HE with low sensitivity to external stimuli such as friction, pressure, temperature, impact, or spark.
According to Samantha Clarke, lead scientist for the experimental work at NIF, the team used the facility’s long laser drive, coupled with two x-ray probe beams on the same target, to produce clear evidence of the formation of products in less than 50 nanoseconds. The experiment captured a time evolution of products under shock compression exceeding 150 gigapascals (1.5 million times Earth’s atmosphere).
This innovative research will inform future development of detection technologies for nonproliferation missions, as well as interdiction technologies for nuclear counterterrorism and other national security missions.
LDRD Project Title: Unlocking the Mysteries of High-Explosive Science
Principal Investigator: Lara Leininger
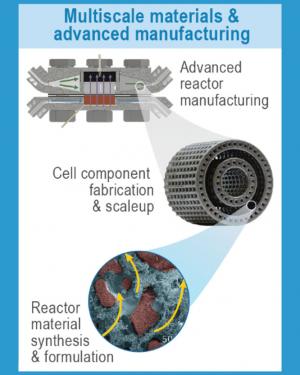
An LDRD-funded team of scientists and engineers developed a new electrochemical reactor paradigm in an effort to fundamentally rethink how chemical reactors are designed, making them smaller, more energy efficient, and more versatile. They also wanted to create an innovative new approach that transforms carbon dioxide (CO2) harvested from the atmosphere into a valuable resource—industrial chemicals, polymers, and other useful compounds.
Much of the chemical-reactor technology in use today involves the use of energy-intensive thermochemical reactors, with an enormous infrastructure and high capital and operating costs. Through several LDRD research projects, including one that wrapped up recently, investigators developed a prototype electrochemical reactor that uses CO2 as the reactant and converts it into multi-carbon molecules, such as ethylene and ethanol. It is small (with an electrode that measures just 1 square centimeter), and it uses a modular platform that can scale up for industrial settings.
The research team integrated computational design, engineering, and materials science to refine the reactor model, along with three-dimensional additive manufacturing techniques, to produce reactor components that offer the microstructure needed to control the catalyst environment. Leveraging the versatility and precise control offered by the additive manufacturing techniques, they can speed up the process to produce electrochemical cells and reactors, and tailor them to specific operational variables.
LLNL materials engineer Eric Duoss and chemist Sarah Baker led the LDRD-sponsored research team that developed the reactor design and engineered the prototype. Baker is also partnering with Duoss to investigate scale-up of the technology through industry and university collaborations.
The smaller, customizable reactor design will enable a transition from expensive, large-scale chemical plants to distributed, more economical chemical reactor networks.
LDRD Project Title: Manufacturing Molecules for the Carbon Economy
Principal Investigators: Eric Duoss and Sarah Baker
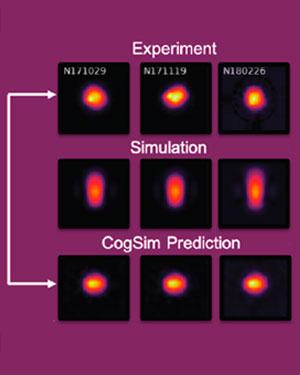
Predictive simulation capabilities play a key role in many high-priority missions, including nuclear security, critical infrastructure protection, and biosecurity. The accuracy of these predictive models relies on the ability to compare simulation results with experimental data. However, in many of these mission-critical research areas, it has been extremely challenging to incorporate the full scope of available experimental data into predictive models.
In an LDRD-funded project, which is part of the Laboratory’s cognitive simulation initiative, researchers are developing advanced machine-learning techniques that will combine our rapidly expanding simulation capabilities with our precision empirical data sets. These learning-based predictive techniques can better align simulations with experimental data, improving the accuracy and efficiency of simulations.
According to Brian Spears, an LLNL physicist who leads the research team, investigators have developed new deep-neural-network technologies designed to make full use of available data sets from experiments. The novel technology is capable of handling extensive collections of multimodal experimental data, including images, vector-valued data, time histories, and scalar measurements.
These transformative predictive capabilities can speed up extremely complex calculations and compare varied data sources efficiently, without requiring a scientist to scan tremendous amounts of data. The interdisciplinary team that developed this exciting new technology includes experts in machine-learning architectures, deep learning, data harvesting, and intelligent data sampling.
“The ability to combine multiple, scientifically relevant data streams will open the door to a wide range of new types of analyses,” explained Spears. “It will allow us to extract information from our most valuable experimental and simulation data sets that has been inaccessible until now. Fully exploiting this information, in concert with a new suite of related cognitive simulation tools, will lead to improved predictive models.”
These new predictive capabilities based on machine learning have already been integrated into multiple programs at LLNL, ranging from atmospheric release modeling to pathogen spread modeling.
LDRD Project Title: Learning-Based Predictive Models: A New Approach to Integrating Large-Scale Simulations and Experiments
Principal Investigator: Brian Spears
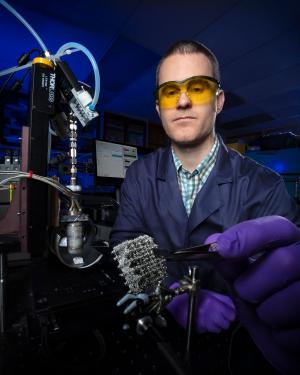
The ability to engineer mission-critical metal parts using advanced manufacturing techniques continues to play a key role in research at LLNL. Existing three-dimensional (3D) printing methods, which build precise parts in sequential layers, do not work well with all types of metals. Recognizing the need to design and deliver metal parts with tailored properties, including parts made from actinide materials, a research team at Livermore adapted a 3D-printing technique known as liquid metal jetting (LMJ) and explored how it can be used to produce parts with the structure and tailored properties needed to meet the needs of national security stakeholders.
According to LLNL physicist Jason Jeffries, who led the LDRD-funded research team, LMJ eliminates problems typically found with selective laser melting (SLM) techniques. “Selective laser melting limits the types of metal feedstocks that can be used because many metals become highly reactive when they are converted to a powder,” explained Jeffries. In addition, the use of fine powders can cause spattering, which may inadvertently contaminate a part, or the powder can get trapped inside a part and vaporize, resulting in an unwanted void in the finished build.
“Liquid metal jetting is a much gentler and more localized process,” said Jeffries. And because LMJ uses raw feedstock, it can bypass the extra processing step of converting it into powder.
LLNL’s multidisciplinary effort to enhance LMJ capabilities included researchers in physics, materials science, mechanical engineering, and computational engineering. They explored how to control tiny droplets of metals, heated above their melting temperatures, which subsequently fall onto a substrate to produce 3D parts from a stack of two-dimensional digital patterns. Upon solidification, each layer of jetted droplets acts as a new substrate onto which new droplets are dispensed to create the adjacent layer. As the droplet-on-demand process repeats, complex 3D geometries can be printed with extremely fine detail.
The team studied how various techniques affect the build speed and quality of parts. For example, they developed and tested a machine with two different printing modes—a continuous stream and a droplet-on-demand process, which produces discrete droplets ejected from a nozzle. By combining the capabilities into a single machine, an object can be manufactured using both modes, offering the speed of a continuous material stream, and the resolution offered by the droplet-on-demand mode.
The team also studied how to modify the process to obtain optimal mechanical properties. For example, they analyzed how much of the underlying structure should melt as the molten drop bonds to the metal underneath it. In addition, they explored ways to control the cooling rate of the microstructure by modifying the droplet ejection velocity, size, and frequency.
Researchers demonstrated that the technique can be used to produce metal parts with unique shapes, structures, and properties that are not possible with conventional machining, casting, or 3D-printing methods.
The LDRD-sponsored research advanced the state-of-the-art in advanced manufacturing with actinides and other metals, improved scientists’ understanding of actinide alloys and high-temperature actinide synthesis, and enhanced LLNL expertise in actinide science.
LDRD Project Title: Developing and Characterizing New Tools for Actinide Processing Science
Principal Investigator: Jason Jeffries
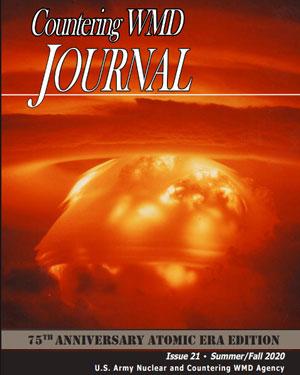
The summer of 1945 marked a turning point in history, with the world’s first nuclear detonations. When editors of a U.S. Army journal decided to publish a special edition commemorating the 75th anniversary of these historic events, they contacted LLNL nuclear forensic scientist Kim Knight, seeking possible articles for a special issue of the Countering Weapons of Mass Destruction (WMD) Journal.
Knight has been studying historic fallout from near-surface nuclear events for many years, and at the time the editors contacted her, she was already working with more than 25 LLNL investigators on an LDRD-funded research initiative studying how different environments affect nuclear explosions.
When the special edition of the journal was released in late 2020, 5 of its 15 articles were written by LLNL scientists.
“The timing of our work fit perfectly for their request,” said Knight. “It was a great way to bring awareness of our work to a broad audience, including our hypothesis that interaction of the local environment with a nuclear detonation changes how the fireball evolves.”
Knight’s research team combined computational and experimental approaches to create an informed framework capable of modeling nuclear detonations, taking into account how the environment contributes to the physical and chemical evolution of the fireball plasma and resultant debris.
Investigators analyzed historical films of nuclear tests and studied nuclear debris; modelers built into simulations of nuclear detonations the key physics and chemistry needed to account for time-dependent entrainment; and experimentalists studied chemical speciation and early debris-formation processes through laser interactions with matter and plasma chemistry.
“The experimental work informs the chemistry that we need for the models, which we’re grounding in historical data from films and debris,” Knight explained.
The research continues to play a key role in expanding LLNL expertise in fallout science, while supporting NNSA’s nuclear threat reduction goal.
LDRD Project Title: Identifying the Influence of Environmental Effects on Post-Detonation Chemistry and Debris Formation
Principal Investigator: Kim Knight
Scientific Leadership and Service
LDRD projects are distinguished by their mission-driven creativity. LDRD-funded research often launches stellar careers, initiates strategic collaborations, produces game-changing technical capabilities, and even lays the foundation for entirely new fields of science. It is no surprise that every year, LDRD principal investigators from LLNL are recognized for the groundbreaking results of a project or long-term contributions to their fields. The following examples highlight recognition received during fiscal year 2020, attesting to the exceptional talents of these researchers and underscoring the vitality of Livermore’s LDRD program.
Fellows
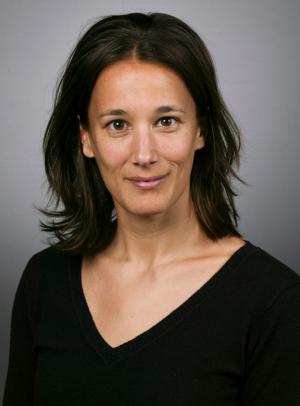
Félicie Albert
Kavli Fellow, National Academy of Sciences
As a new elected Kavli fellow of the U.S. National Academy of Sciences, Félicie Albert was invited to present a poster at the annual U.S. Kavli Frontiers of Science symposium, where she discussed her research on using plasmas produced by intense lasers as particle accelerators and x-ray light sources. Albert currently serves as the deputy director of LLNL’s High Energy Density Science Center. She is a fellow of the American Physical Society and a senior member of the Optical Society of America.
“I am really honored to have been selected as a Kavli fellow and to have been invited to the U.S. Kavli Frontiers of Science Symposium. The event was a great way to showcase the work we do at LLNL using lasers.”
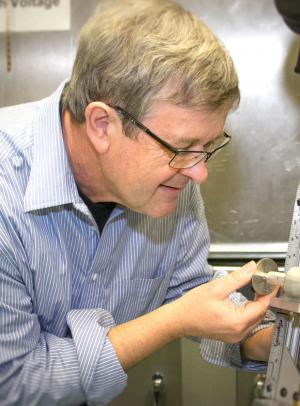
Peter Beiersdorfer
Fellow, American Astronomical Society
Peter Beiersdorfer’s selection as a fellow of the American Astronomical Society (AAS) builds on the recognition he received with his previous Laboratory Astrophysics Prize from AAS. During his career, Beiersdorfer has pioneered techniques to reproduce conditions in the sun’s atmosphere, interstellar space, the centers of galaxies, and on comets. A major focus of his research is characterizing atomic and molecular diagnostics as revealed by their x-ray spectra. His studies of emissions from the inner electron shells of iron, oxygen, neon, silicon, and sulfur are used to interpret the physical conditions in astronomical environments near and far. His work on x-ray emission from charge exchange revealed the importance of this process in cometary atmospheres.
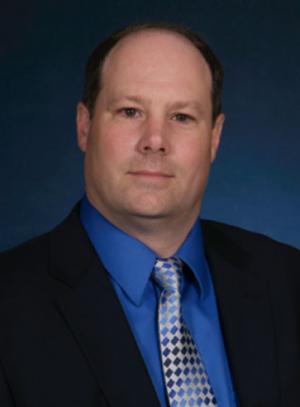
J. Chance Carter
Fellow, Society of Applied Spectroscopy
Chance Carter was selected as a fellow of the Society of Applied Spectroscopy for his exceptional contributions to spectroscopy. His achievements include development of spectroscopic-based analytical methods and systems, remote and standoff Raman and infrared spectroscopy, and fiber-optic sensors.
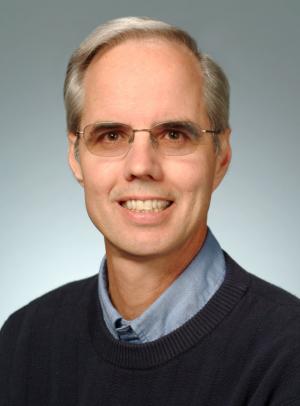
William Pitz
Fellow, Society of Automotive Engineering
The Society of Automotive Engineering selected physicist William (Bill) Pitz as a fellow in recognition of his significant impact on the development of mobility technology through leadership, research, and innovation. His research at LLNL includes studies of combustion phenomena in various types of engines.
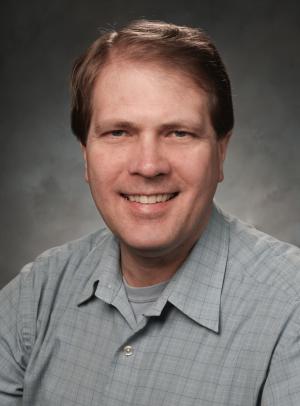
Bronis de Supinski
Fellow, Institute of Electrical and Electronics Engineers
The Institute of Electrical and Electronics Engineers named Bronis de Supinski as a fellow in recognition of his leadership in the design and use of large-scale computing systems. As chief technology officer for Livermore Computing, he is responsible for formulating LLNL's large-scale computing strategy and overseeing its implementation. His research interests include compilers, tools, and runtime systems, particularly programming models. He also chairs the OpenMP Language Committee.
“I am pleased to be elevated to an IEEE fellow. I am grateful to my colleagues for their essential contributions to the research and system development that are the hallmark of my achievements. These achievements have enabled me to reach a goal that I set for myself many years ago—to be a world-leading computer scientist.”
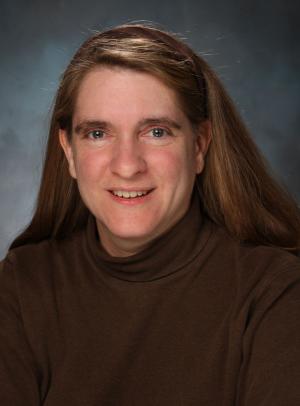
Carol Woodward
Fellow, Association for Women in Mathematics
The Association for Women in Mathematics (AWM) named computational scientist Carol Woodward a fellow, recognizing her commitment to supporting and advancing women in the mathematical sciences. A computational mathematician in LLNL’s Center for Applied Scientific Computing since 1996, Woodward’s research focuses on nonlinear solvers and time-integration methods and software. She is part of the DOE FASTMath SciDAC Institute project to improve numerical software for use in DOE applications. She is developing integration methods for transmission power grid simulation as part of DOE’s advanced grid modeling program and for climate simulations as part of the greater SciDAC program.
“Being selected as an AWM fellow is special to me. Promoting the amazing work that women do in mathematics, as well as encouraging equal treatment for women, have been causes I strongly support and have worked hard to develop. The AWM does so much amazing work in support of women and girls in mathematics, and I find it a humbling honor to be recognized by them.”
Other Awards
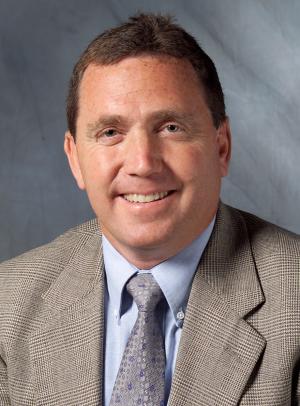
Bert Bolin Award, American Geophysical Union
Atmospheric scientist Benjamin Santer was honored with the American Geophysical Union’s 2020 Bert Bolin Award, recognizing groundbreaking research or leadership in global environmental change. Santer’s work focuses on climate model evaluation, the use of statistical methods in climate science, and the identification of natural and anthropogenic "fingerprints" in observed climate records. His early research on the climatic effects of combined changes in greenhouse gases and sulfate aerosols contributed to the historic "discernible human influence" conclusion of the 1995 IPCC report. His recent work attempts to identify anthropogenic fingerprints in a number of climate variables, such as tropopause height, atmospheric water vapor, the temperature of the stratosphere and troposphere, oceanic heat content, and ocean-surface temperatures in hurricane formation regions.
“This award has deep personal meaning for me. I’ve never forgotten Bert Bolin’s kindness and encouragement. The lecture will be an opportunity to pay tribute to a great man and a great scientist.”
John Dawson Award, American Physical Society
Three LLNL scientists received the 2020 John Dawson Award for Excellence in Plasma Physics Research from the American Physical Society. The team generated Weibel-mediated collisionless shocks in the laboratory, informing a broad range of energetic astrophysical scenarios, plasma physics, and experiments that use high-energy and high-power lasers at plasma science facilities.
Collisionless shocks have been of intense scientific interest for more than half a century. They are a fixture in astrophysical plasmas and are believed to generate and amplify magnetic fields in the universe and accelerate particles as a source of cosmic rays in a variety of objects, including colliding galaxies, supernova explosions, and gamma-ray bursts.
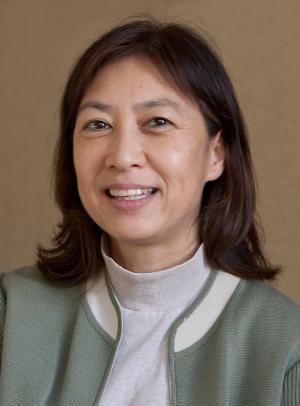
Hye-Sook Park
“It is a great honor to receive this award. I truly appreciate all the team members and the fact that our devotion to great science has been recognized. I am thrilled to be a part of the work that solved a small piece of the puzzle of supernova explosions.”
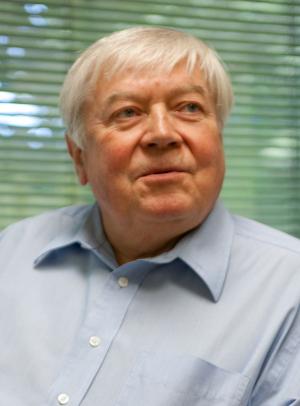
Dmitri Ryutov
“Being a theorist, I enjoy working on projects where theory is closely coupled to an ongoing experiment and helps in the decision making. It was a great privilege for me to work with outstanding LLNL experimentalists. We have made one more step toward a better understanding of the processes driven by supernova explosions and other energetic events occurring in the universe.”
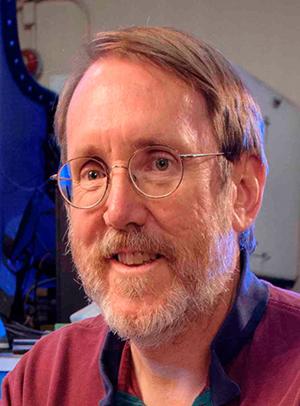
James Steven Ross
“The idea of generating collisionless shocks was proposed ten years ago. It took a combination of improved physics understanding and new diagnostic capabilities to make this project successful. I find it very exciting that we can use the world’s largest laser to create centimeter-scale plasmas that are relevant to astrophysical phenomena spanning light years.”
DOE Office of Science Early Career Research Program Award
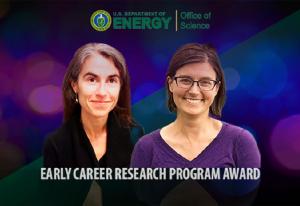
Two scientists who started their careers at LLNL as postdoctoral researchers and went on to lead LDRD-sponsored research received awards this year from the DOE Office of Science Early Career Research Program. The program is designed to bolster the nation’s scientific workforce by providing support to exceptional researchers during crucial early career years, when many scientists do their most formative work. Under the program, DOE national laboratory staff are awarded $500,000 per year for five years to further their research.
Physicist Federica Coppari, whose work on large laser facilities helped lead to the discovery of the atomic structure of superionic ice, was one of this year’s award recipients. Coppari joined the Lab in 2011 as a postdoctoral researcher, and today she works on developing new experimental platforms to understand material behavior at extreme conditions, with a focus on the physics of phase transitions, melting, and material equations of state.
Giant lasers can compress and heat matter to extreme states. When combined with bright x-ray sources, they become very powerful tools for investigating the atomic-level structural changes induced by strong and ultrafast compression. Coppari’s experiments are conducted in facilities such as LLNL’s National Ignition Facility and at synchrotrons, where she uses ultrafast x-ray diffraction and extended x-ray absorption fine-structure diagnostics to characterize material properties at extreme conditions.
Coppari plans to use the DOE funding to investigate mixing and metastability in warm dense matter, with an emphasis on planetary constituent materials. “Matter deep inside planets is at extreme pressures and temperatures, and knowledge of its properties is key to improving our understanding of planetary formation and interior structure,” she said. “It is extremely important to know how the different constituents mix (or unmix) when subjected to extreme conditions, although this is far from being understood.”
“Pulling together ultrafast compression with x-ray and optical diagnostics, my research will investigate the transformations happening at extreme conditions of pressure, temperature, and timescale in complex, multi-component systems to obtain a better understanding of their mixing properties and pathways to phase transitions,” Coppari explained.
Microbiologist Erin Nuccio was selected for her research in fundamental systems biology, including her work studying the role of microbes in bio-geochemical cycling processes using a systems biology approach.
Nuccio started at LLNL in 2013 as a postdoc studying plant-microbe interactions. She has served as a staff scientist since 2016, and is now studying how microbes control carbon and nutrient cycling in soil, with a focus on the rhizosphere and hyphosphere. She uses stable isotopes to track interactions and nutrient exchanges in the complex soil environment.
“Throughout my career, I have been keenly interested in the fundamental ecology of plant-microbe-soil interactions, and the role these relationships play in terrestrial carbon cycling and ecosystem sustainability,” she said.
According to Nuccio, microbes engage in a lively ‘cross-talk’ that we are only beginning to understand through next-generation sequencing and metabolomics technologies. She looks forward to digging deeper into the fungal hyphosphere—an active site of soil nutrient cycling that we know little about, although it is a hotspot for fungal-bacterial interactions. For fungi that cannot decompose plant material by themselves, this is a key zone for nutrient foraging. Nuccio expects fungal interactions with the microbial community to be of paramount importance in this region.
“It’s an incredible honor to be selected for this award. It is a game-changer for building my research career at LLNL.”
Vaccine Delivery Platform Adapted to Fight Biothreats and Other Dangerous Pathogens
Biotechnology developed through a series of LDRD-funded projects is now poised to serve as a versatile platform for drug and vaccine delivery across a range of health challenges. Initially developed to study membrane protein function, this tiny, yet highly powerful nanotechnology is being used to defend against biological threats. It is also being adapted to serve as a potent, safe, targeted vaccine delivery platform.
Tailored nanoparticle platform delivers customized vaccines
The biotechnology got its start in 2005 with a three-year LDRD-funded research project led by LLNL chemist Paul Hoeprich. According to Hoeprich, his research team explored ways to leverage research in cell membrane biochemistry to better understand the mechanics of drug transport into cells. “We brought these ideas together, developing our initial concept for a nanoscale drug delivery platform,” said Hoeprich.
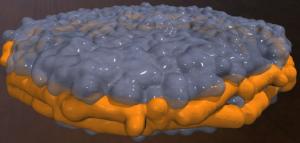
During several LDRD-funded projects spanning more than a decade, investigators developed and tested the nanolipoprotein particle (NLP) platform, which can be tailored to activate the immune system against multiple pathogens. The platform’s foundation consists of naturally occurring molecules that mimic cell membranes. They can self-assemble, similar to interlocking building blocks, and provide a platform for connecting other biomolecules.
For example, scientists can attach adjuvants (molecules designed to boost vaccine potency) and antigens (molecules capable of inducing a specific immune response) to an NLP platform, making it possible to administer both types of molecules in a single controlled package aimed at responding to a specific type of pathogen. The disc-shaped platform measures between 8 and 25 nanometers in diameter, making it the ideal size to leverage natural pathways into cells, particularly immune cells relevant to vaccine delivery.
Rather than serving as a single-pathogen solution, the NLP platform provides the foundation to fabricate multiple types of vaccines. And since the particles are naturally present in the human body, vaccines produced using the NLP platform are less likely to result in toxicity.
Biodefense solutions
The initial focus of LLNL’s research regarding NLP-based vaccines was their potential to protect military personnel and first responders against biothreat pathogens with no existing vaccines, or vaccines that require multiple doses to elicit a protective immune response.
As the technology matured through these initial LDRD investments, additional funding provided by the U.S. Defense Threat Reduction Agency (DTRA) enabled LLNL scientists to focus on ways they could use NLP technology to develop a vaccine capable of responding to one of the most infectious bacterial pathogens in existence, Francisella tularensis, the bacterium that causes tularemia. Although the pathogen is rare, it has a high mortality rate, even at low doses. It is considered to be a potential biothreat agent based on its extremely low infectious dose.
LLNL investigators are collaborating with scientists from the University of New Mexico to develop a multi-antigen vaccine capable of stimulating strong antibody and T-cell responses, providing protection against the bacteria, even when aerosolized. It uses the NLP delivery platform to co-deliver the tailored combination of antigens and adjuvants. They recently expanded their collaboration to include Tulane University as they explore ways to optimize the tularemia vaccine for clinical use and produce it at scale.
Similarly, in 2011, the National Institutes of Health (NIH) provided funding for a collaborative research initiative, co-led by LLNL and Loyola University, aimed at developing an improved vaccine to protect against Bacillus anthracis, the causative agent of anthrax.
Public health applications
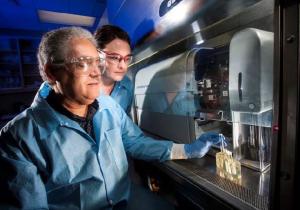
Biotechnology experts at LLNL continue to fine-tune the NLP technology, recognizing its potential to address other types of pathogens. For example, in 2016, with NIH funding, LLNL scientists collaborated with researchers at the University of California (UC), Irvine, to explore how NLP technology could be used to develop a vaccine that provides protection against chlamydia, the most common sexually transmitted pathogen in the world.
Building on this initial work, in 2019, NIH provided additional funding to establish a cooperative research center and expand these efforts. LLNL leads the center, which includes a multidisciplinary team of experts in immunology and nanotechnology from LLNL, UC Irvine, and UC Davis. Together, they are exploring the most promising antigen formulas, as well as how to refine the NLP platform to effectively deliver the chlamydia vaccine.
Coronavirus vaccine development
The most recent application NLP technology involves the search for a broad-spectrum, universal coronavirus vaccine, capable of providing protection against coronavirus pathogens such as Middle East Respiratory Syndrome (MERS), Severe Acute Respiratory Syndrome (SARS), and SARS-CoV-2, the virus that causes COVID-19. With an LDRD investment in this new research, which launched in 2020, LLNL investigators are collaborating with ConserV Bioscience, a vaccine development company.
A broad-spectrum vaccine is a necessary next step to protect against continued mutations of SARS-CoV-2, as well as new coronavirus strains that become more virulent and pose a pandemic threat. The team will focus on designing a vaccine that targets regions of the virus proteins that are not susceptible to change, enabling it to provide protection as the virus mutates. In addition, researchers anticipate that the NLP platform will reduce the timeframe needed to develop new vaccines.
Timeline
2005 | LDRD investments fund initial explorations of a new biotechnology to enhance vaccine development and delivery. |
2011 | LLNL investigators start exploring ways to use NLP technology to protect against biothreats and deliver cancer therapeutics. |
2014 | LLNL starts exploring use of NLP technology for subunit vaccines, collaborating with Synthetic Genomics, a biotech company, to produce vaccines based on embedded membrane proteins and self-replicating mRNA. |
2016 | NIH funding supports development of a chlamydia vaccine using NLP technology. Follow‑on NIH funding in 2019 established a cooperative research center. |
2017 | NLP technology licensed by EVOQ Therapeutics for cancer immunotherapy, leveraging the platform’s capabilities to deliver personalized vaccines to patients’ lymph nodes and activate the immune system to attack cancer cells. |
2020 | DTRA-funded research begins, focused on using NLP technology to develop and optimize a tularemia vaccine. |
2021 | LLNL initiates an industry collaboration to explore how NLP technology can be used to develop a broad‑spectrum vaccine against coronavirus pathogens. |
Related LDRD Projects
- PI Paul Hoeprich (Project 06-SI-003)
- PI Craig Blanchette (Projects 09-LW-007 & 15-LW-023)
- PI Amy Rasley (Project 11-ERD-016)
- PI Nicholas Fischer (Projects 11-LW-015 & 20-ERD-004)
- PI Matthias Frank (Project 12-ERD-031)
- PI Sean Gilmore (Project 17-LW-051)