Program Value
By almost any measurement, the LDRD program contributes far more in publications, intellectual property, collaborations, and recruitment of postdoctoral researchers—dollar for dollar—than any other program at the Laboratory.
- Collaborative Explorations
- Intellectual Property
- Scientific Publications
- Early Career
- Professional Fellows
- Long-term Impact
Collaborative Explorations
External collaborations are essential to the innovative research that takes place at LLNL, including LDRD-funded projects. By collaborating with other national laboratories, academia, and industry, our investigators can engage with experts from other institutions and access world-class experimental facilities.
The following table provides our most recent data regarding formal collaborations, which we define as LDRD-funded projects where an external collaborator received LDRD funds from LLNL. In addition, our investigators frequently participate in informal collaborations with researchers at other institutions, which often involves joint scientific publications. Both types of collaborations are a key indicator of the broad intellectual engagement that is a hallmark of LLNL’s research environment.
66 INSTITUTIONS were involved in formal collaborations with LLNL as part of LDRD-funded research teams in FY22.
Collaborations | FY18 | FY19 | FY20 | FY21 | FY22 |
---|---|---|---|---|---|
LDRD-funded projects with one or more formal collaborations | 74 | 74 | 78 | 88 | 79 |
Percentage of all projects at LLNL | 31% | 30% | 32% | 33% | 32% |
New laser-based volumetric additive manufacturing method can 3D print glass in seconds
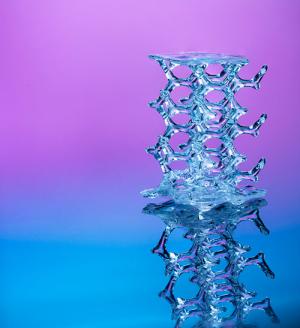
Versatile and ubiquitous, glass is increasingly found in specialized applications such as fiber optics, consumer electronics and microfluidics for “lab-on-a-chip” devices. However, traditional glassmaking techniques can be costly and slow, and 3D-printing glass often results in rough textures, making them unsuitable for smooth lenses.
Using a new laser-based Volumetric Additive Manufacturing (VAM) approach — an emerging technology in near-instant 3D printing — researchers at Lawrence Livermore National Laboratory (LLNL) and the University of California, Berkeley have demonstrated the ability to 3D-print microscopic objects in silica glass, part of an effort to produce delicate, layer-less optics that can be built in seconds or minutes. The results are reported in the latest edition of the journal Science.
Nicknamed “the replicator” after the fictional device in “Star Trek” that can instantly fabricate nearly any object, the Computed Axial Lithography (CAL) technology developed by LLNL and UC Berkeley is inspired by computed tomography (CT) imaging methods. CAL works by computing projections from many angles through a digital model of a target object, optimizing these projections computationally and then delivering them into a rotating volume of photosensitive resin using a digital light projector. Over time, the projected light patterns reconstruct, or build up, a 3D light dose distribution in the material, curing the object at points exceeding a light threshold while the vat of resin spins. The fully formed object materializes in mere seconds — far faster than traditional layer-by-layer 3D printing techniques — and then the vat is drained to retrieve the part.
Combining a new microscale VAM technique called micro-CAL, which uses a laser instead of an LED source, with a nanocomposite glass resin developed by the German company Glassomer and the University of Freiburg, UC Berkeley researchers reported the production of sturdy, complex microstructure glass objects with a surface roughness of just six nanometers with features down to a minimum of 50 microns.
UC Berkeley Associate Professor of Mechanical Engineering Hayden Taylor, the project’s principal investigator, said the micro-CAL process, which produces a higher dose of light and cures 3D objects faster and at higher resolution, combined with the nanocomposite resins characterized at LLNL proved a “perfect match for each other,” creating “striking results in the strength of the printed objects.”
LLNL co-author Caitlyn Krikorian Cook, a group leader and polymer engineer in the Lab’s Materials Engineering Division, characterized the curing kinetics of the nanocomposite resin with light exposure. Printing higher-viscosity resins is challenging, if not impossible, with current traditional stereolithography systems at LLNL, according to Cook, and that the benefit of VAM for micro-optics is that it can produce extremely smooth surfaces without layering artifacts, resulting in faster printing without additional post-processing time.
“You can imagine trying to create these small micro-optics and complex microarchitectures using standard fabrication techniques; it’s really not possible,” Cook said. “And being able to print it ready-to-use without having to do polishing techniques saves a significant amount of time. If you can eliminate polishing steps after forming the optics — with low roughness — you can print a part ready for use.”
Cook performed in-situ resin characterization with a spectrometer to measure the thresholding response of an inhibitor modifier in the material’s photopolymerization kinetics. The modifier, combined with the preciseness of the laser VAM method, was the “secret sauce” to printing high-resolution optics at a microscale.
“By creating a thresholding response, we’re able to significantly improve the resolution,” Cook said. “We’re taking advantage of the similar thresholding response reported in our previous work, except we’re implementing it in a different class of photopolymer chemistry. We’re beginning to better understand the necessary kinetics for volumetric manufacturing.”
Real-world applications could include micro-optics in high-quality cameras, consumer electronics, biomedical imaging, chemical sensors, virtual-reality headsets, advanced microscopes, and microfluidics with challenging 3D geometries such as “lab-on-a-chip” applications (where microscopic channels are needed for medical diagnostics), fundamental scientific studies, nanomaterial manufacturing and drug screening. Plus, the benign properties of glass lend themselves well to biomaterials, or to cases with high temperature or chemical resistance.
LDRD Project Title: Advanced Photopolymer Materials Engineering for Multiscale Additive Fabrication
Principal Investigator:
Maxim Shusteff
LDRD Project:
19-ERD-012
Intellectual Property
Year after year, projects sponsored by LDRD achieve a disproportionately large percentage of the patents and copyrights issued for LLNL research. As illustrated in the following tables, LDRD-funded work has been key in developing more than half of the Laboratory’s patents, one-third of the Laboratory’s copyrights (chiefly computer code), and more than half of the Laboratory’s records of invention.
LDRD-funded work has played a key role in developing MORE THAN 50% of the Laboratory’s patents.
Patents | FY18 | FY19 | FY20 | FY21 | FY22 |
---|---|---|---|---|---|
All LLNL patents | 79 | 143 | 200 | 166 | 166 |
LDRD patents | 41 | 95 | 131 | 96 | 103 |
LDRD patents as a percentage of total | 52% | 66% | 66% | 58% | 62% |
Copyrights | FY18 | FY19 | FY20 | FY21 | FY22 |
---|---|---|---|---|---|
All LLNL copyrights | 105 | 118 | 138 | 125 | 142 |
LDRD copyrights | 23 | 24 | 31 | 42 | 47 |
LDRD copyrights as a percentage of total | 22% | 20% | 22% | 34% | 33% |
Records of Invention | FY18 | FY19 | FY20 | FY21 | FY22 |
---|---|---|---|---|---|
All LLNL records | 105 | 129 | 126 | 89 | 70 |
LDRD records | 47 | 65 | 56 | 53 | 40 |
LDRD records as a percentage of total | 45% | 50% | 44% | 60% | 57% |
LLNL team claims top AI award at international symbolic regression competition
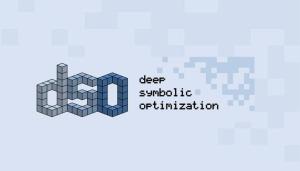
A Lawrence Livermore National Laboratory (LLNL) team claimed a top prize at an inaugural international symbolic regression competition for an artificial intelligence (AI) framework they developed capable of explaining and interpreting real-life COVID-19 data.
Hosted by the open source SRBench project at the 2022 Genetic and Evolutionary Computation Conference (GECCO), the competition had two tracks — synthetic and real-world — and invited teams to submit their best symbolic regression algorithms. Organizers trained the models on datasets, assigned “trust ratings” and evaluated them for accuracy and simplicity.
LLNL computer scientist Brenden Petersen and his team’s “Unified Deep Symbolic Regression” (uDSR) algorithm beat out 12 other teams on the real-world track — a task to build an interpretable predictive model for 14-day forecast counts of COVID-19 cases, hospitalizations, and deaths in New York state.
The team’s uDSR method is an updated version of their earlier deep symbolic regression algorithm — a reinforcement learning approach using deep neural networks — that finds short mathematical expressions to best fit experimental data and uncovers underlying equations or dynamics of physical processes. The initial framework outperformed previous state-of-the-art baseline methods on benchmark problems. Researchers said the new version unifies deep symbolic regression with four other classes of state-of-the-art symbolic regression algorithms, hybridizing their key capabilities into a single, modular framework.
uDSR is part of a larger framework called Deep Symbolic Optimization (DSO), developed by the team as part of the LDRD. DSO is now being used in several other programs at LLNL: for example, to optimize antibody sequences to bind emerging pathogens.
“This competition is a big win for the Laboratory because our underlying DSO framework is being used for several Lab missions, not just symbolic regression,” said Petersen, who serves as the principal investigator on the project. “This victory establishes that we are supporting several Laboratory programs with bleeding-edge technologies of a highly competitive field.”
LDRD Project Titles: Hypothesis Testing via Artificial Intelligence: Generating Physically Interpretable Models of Scientific Data with Machine Learning
Principal Investigator:
Brenden Petersen
LDRD Project:
19-DR-003
Scientific Publications
Laboratory scientists and engineers publish more than a thousand papers each year in a wide range of peer-reviewed journals, of which LDRD-funded work accounts for a large portion. The numerous publications made possible through LDRD-sponsored research help the Laboratory maintain a strong presence in the broader scientific community, extending the impact of LDRD research beyond the DOE mission space into the wider scientific arena. In addition, the impact of these publications documenting LDRD project results extends long after articles appear in the journals, increasing the value of LDRD investments in these projects.
Journal Articles | FY18 | FY19 | FY20 | FY21 | FY22 |
---|---|---|---|---|---|
All LLNL articles | 1,178 | 1,281 | 1,149 | 1,256 | 1,149 |
LDRD articles | 456 | 553 | 428 | 509 | 490 |
LDRD articles as a percentage of total | 39% | 43% | 37% | 41% | 43% |
New method sheds light on solid-state battery fabrication
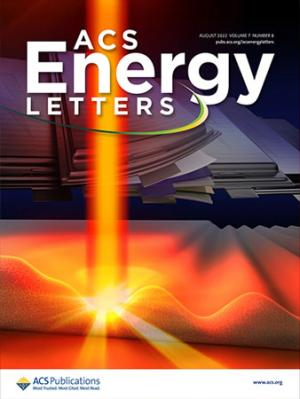
Safe, long-cycle-life batteries with high energy density are greatly needed with the rapid growth of electric devices and vehicles and grid energy storage demands. Lawrence Livermore National Laboratory (LLNL) scientists have devised a method for the fabrication of all-solid-state lithium metal batteries, which have been recognized as the future choice of safe and high-energy-density power sources.
The team found that sintering (compacting and forming a solid mass of material by heat or pressure without melting it to the point of liquefaction) the solid-state electrolyte films with carbon dioxide (CO2) overcomes the common manufacturing challenges in solid-state batteries.
The laser sintering technique yields scalable, low-cost, high-energy-density solid-state lithium (Li) batteries that can advance energy storage needs across national security missions. These laser-based techniques also can be applied to other fields, such as additive manufacturing of polymers, ceramics, and metals.
Typical Li-ion batteries (LIBs) use organic liquid electrolytes and graphite anodes, which lead to safety hazards and lower the energy density. Among the various substitutes, all-solid-state Li-metal batteries — with the integration of solid-state electrolytes (SSEs) — are foreseen as the next generation of energy-storage devices that not only effectively address battery safety concerns, but also enable the utilization of Li metal anodes and high-capacity/high-voltage cathode materials to achieve higher energy density.
“This work provides a unique, scalable and widely applicable ultrarapid laser sintering technique to overcome the difficulties associated with classic methods for the integration of SSEs for practical solid-state Li-metal battery applications," said LLNL materials scientist Jianchao Ye, corresponding author of a paper appearing on the cover of ACS Energy Letters.
LDRD Project Title: Laser Processing of Solid State Lithium Batteries
Principal Investigator:
Jianchao Ye
LDRD Project:
20-ERD-018
Early Career Opportunities: Students and Postdoctoral Fellows
By funding exciting, potentially high-payoff projects at the frontiers of science, the LDRD program attracts top talent in new and emerging fields of science and technology. As shown in the following tables, LDRD investments contribute to the health and robustness of LLNL’s student and postdoctoral researcher programs.
Students | FY18 | FY19 | FY20 | FY21 | FY22 |
---|---|---|---|---|---|
Students supported by LDRD | 138 | 160 | 101 | 136 | 149 |
Percentage of all students | 22% | 23% | 18% | 24% | 23% |
Postdoctoral Researchers | FY18 | FY19 | FY20 | FY21 | FY22 |
---|---|---|---|---|---|
Postdoctoral researchers supported by LDRD ≥10% of their time | 167 | 170 | 208 | 208 | 240 |
Percentage of all postdoctoral researchers | 54% | 57% | 63% | 54% | 56% |
LDRD postdoctoral researchers converted to full staff | 52 | 46 | 60 | 50 | 72 |
Percentage of all conversions | 71% | 68% | 77% | 82% | 77% |
Cancer therapies and nuclear material detection get a boost from newly discovered protein
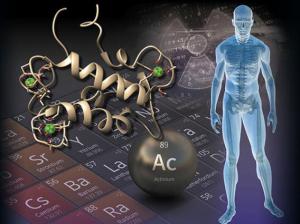
Lawrence Livermore National Laboratory (LLNL) and Penn State scientists have demonstrated how a protein can be recovered and purified for radioactive metals like actinium that could be beneficial for both next-generation drugs used in cancer therapies and the detection of nuclear activities.
Radioactive metals hold unique and essential places in a variety of medical imaging and therapeutic applications, but they require lengthy separation processes for their purification as well as synthetic and costly chelators (molecules tailored to bind the radioactive metal ions) that must form exceptionally stable complexes in the patient to minimize toxicity. Actinium-based therapies could revolutionize cancer medicine, with treatment efficacy hundreds of times higher than current drugs. However, they remain out of reach due to difficulties throughout the actinium supply chain, from isotope production to studying the element’s chemistry, and development of robust chelators for it.
“These challenges exist even for medical isotopes in relatively widespread use, such as radioactive yttrium, but they are even more taxing in the case of actinium,” said LLNL scientist Gauthier DeBlonde, a Post-Doc at LLNL and lead author of a paper appearing in Science Advances.
As it turns out, actinium is one of the rarest elements naturally occurring on Earth, and its medical isotope must be produced in nuclear reactors or other large and costly instruments. As such, actinium chemistry is among the least understood and this impedes the development actinium-based medical applications. Research efforts have thus far focused on reusing or adapting known synthetic molecules used in the nuclear chemistry field, but results have been limited with actinium. The new research took a drastically new approach and leveraged a natural protein, lanmodulin, discovered by the Penn State members of the team in 2018. This new strategy not only improves and simplifies the purification processes for actinium but can also be used to recover and detect other radioactive elements, even at extremely low levels.
The team showed how lanmodulin can be used to bind, recover, and purify actinium (at least 99.5 percent purity obtained in a single step), as well as another medically relevant radioisotope, yttrium-90, which is used for cancer therapy and diagnostics. The unprecedented efficiency and simplicity of the protein-based approach also allows preparation of actinium at much lower cost and probing its chemistry more conveniently. The process also is likely extendable to many other radioactive isotopes used in radiation therapy and imaging.
“Our new technique represents a paradigm shift not just in the development of actinium chemistry and actinium-based pharmaceuticals, but also in nuclear medicine more generally,” said Joseph Cotruvo Jr., co-corresponding author, and Penn State assistant professor of chemistry.
The actinium-lanmodulin complex is the very first actinium protein to be characterized. The researchers found that lanmodulin is so efficient compared to classic molecules that it specifically binds actinium even in the presence of large quantities of process impurities, like radium and strontium, or physiological elements like calcium, zinc, copper, etc. The study also demonstrates that the protein is more effective at binding actinium than binding the rare earth elements, the metals it binds to in nature.
DeBlonde said, “What we accomplished here was simply unfathomable a few years ago. The unique combination of skills in radiochemistry, metal separation and biochemistry at LLNL and Penn State made this possible. I believe our technology will be incredibly useful not only to nuclear medicine but also for the in-field analysis of radioactive samples.”
LDRD Project Title: Elusive Actinium: A First Glimpse into the Coordination Chemistry of Element 89
Principal Investigator:
Gauthier DeBlonde
LDRD Project:
20-LW-017
Professional Fellows
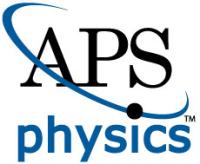
One relevant indicator of advancement and leadership in a scientific field is the election of individuals as fellows of professional societies. This indicator reflects success for both the individual researcher and the Laboratory as a whole.
American Physical Society (APS) fellowships are awarded based on scientific merit and impact over an extended period, and the evaluation process relies on nomination and recommendation by peers. As such, data regarding the history of APS fellowships awarded to LLNL physicists provide an important indicator regarding the key role that the LDRD Program plays in developing the technical, scientific, and leadership skills of early career staff. As presented in the following table, for fiscal year 2022, 100% of the new APS Fellows from LLNL have early career LDRD experience.
Because the quantity of awards each year is a small number, we also present multi-year statistics. For example, over the last 20 years, more than 90% of the APS Fellows at LLNL had early career LDRD experience.
Single-Year Statistics | Multi-Year Statistics | |||||
---|---|---|---|---|---|---|
History of APS Fellows at LLNL | FY20 | FY21 | FY22 | FY13–17 (5 yrs) |
FY18–22 (5 yrs) |
FY13–22 (10 yrs) |
Total APS awards | 4 | 3 | 2 | 30 | 19 | 49 |
Awards with LDRD roots | 4 | 3 | 2 | 29 | 18 | 47 |
% with LDRD roots | 100% | 100% | 100% | 97% | 95% | 96% |
Average years from first LDRD experience | 20.8 | 8 | 15 | 14.8 | 15.9 | 15.2 |
Tracing impact to LDRD roots
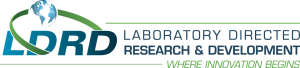
Throughout this section, we mention “LDRD roots.” Much discussion with principal investigators has transpired about what it means for an accomplishment to have LDRD roots. A simple case would be if an idea for an invention arises during an LDRD project and work on the invention is completed during the period of LDRD investment. But R&D often does not advance on such a short timescale. In general, an accomplishment (invention, paper, capability, etc.) is determined to have LDRD roots if at least one LDRD project needed to occur for the accomplishment to take place. In other words, if one can identify an LDRD project that was critical to the accomplishment, then it is considered to have LDRD roots.
2022 APS Fellows at LLNL
Two LLNL scientists were selected as 2022 fellows of the American Physical Society. The new fellows were both selected by the APS Division of Plasma Physics.
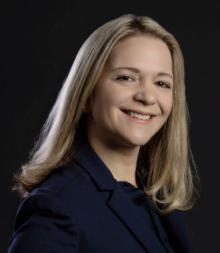
Annie Kritcher
“I am extremely honored to be receiving this award. This is one of the most important awards I will achieve in my career and I’m grateful to have been chosen to become an APS Fellow.”
Annie Kritcher, a physicist in the Weapons and Complex Integration’s Design Physics Division, was selected “for leadership in integrated hohlraum design physics leading to the creation of the first laboratory burning and igniting fusion plasma.” Annie is a team lead within the Inertial Confinement Fusion (ICF) program, where she oversees the integrated modeling of indirect-drive fusion designs fielded at the NIF.
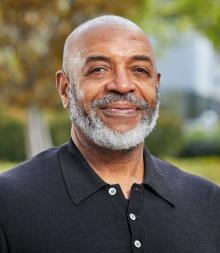
Ronnie Shepherd
“I am honored at being elected an APS-DPP fellow. The honor is a recognition of all the hard work of those that have helped develop the LLNL ultrafast X-ray streak camera, analyze the data, and perform simulations to interpret the physics. I owe them all a great debt of gratitude."
A 38-year veteran, Shepherd serves as the director of outreach at the High Energy Density Science Center. His work focuses on experimental studies of atomic processes in high-density plasmas. The experiments are performed using ultrafast X-ray spectroscopy of short-pulse, laser-heated solids.
Long-term Impact
The LDRD program is an investment in our nation’s future, ensuring mission support that is often realized many years after an LDRD-funded project concludes. Recognizing this long-term impact of the LDRD program, we believe it is important to highlight indicators that span multiple years, demonstrating the true impact of LDRD as a national asset.
We collaborated with our colleagues from LDRD programs at other NNSA institutions to identify ways that we could best represent the long-term impact of LDRD investments. As each institution issues its LDRD program report for fiscal year 2022, we present a common set of long-term performance indicators including the content provided below.
Distinguished Member of the Technical Staff
One relevant indicator of career advancement in a science and technology field is the recognition of individuals as distinguished members of the technical staff at the institution. Individuals who receive this recognition are identified as being in the top 1% or 2% of the institution’s scientific and technical staff, similar to a lifetime achievement award, or in this case, for their contribution to the Laboratory’s mission.
At LLNL, appointment as a Distinguished Member of the Technical Staff (DMTS) is reserved for Laboratory scientists and engineers who have demonstrated a sustained history of high-level achievements in programs of importance to the Laboratory, become a recognized authority in the field, or made a fundamental and important discovery that has sustained, widespread impact.
As presented in the table on the following page, a vast majority of these distinguished staff at LLNL had early career experience with LDRD projects, which helped them develop their scientific, technical, and leadership skills.
Single-Year Statistics | Multi-Year Statistics | |||||
---|---|---|---|---|---|---|
History of DMTS Awards at LLNL | FY20 | FY21 | FY22 | FY13–17 (5 yrs) |
FY18–22 (5 yrs) |
FY13–22 (10 yrs) |
Total DMTS awards | 0 | 0 | 26 | 24 | 36 | 60 |
DMTS with LDRD roots | N/A | N/A | 23 | 21 | 33 | 54 |
% with LDRD roots | N/A | N/A | 89% | 88% | 92% | 90% |
Average years from first LDRD experience | N/A | N/A | 20 | 14.0 | 19.3 | 17.3 |
R&D 100 Awards
Another indicator of advancement and leadership in a scientific field is the R&D 100 Award program, which honors the top innovations of the past year. R&D 100 Awards can occur a long time after the initial ideas are developed, often during LDRD projects. Typically, it takes 5 to 10 years (or longer) from concept development to receiving an award, including the time needed to move through patenting an invention and demonstrating its commercial applications.
The LDRD program is an investment in our nation’s future, ensuring mission support that is often realized many years after an LDRD-funded project concludes. Recognizing the is long-term impact of the LDRD program, we believe it is important to highlight indicators that span multiple years, demonstrating the true impact of LDRD as a national asset.
Over the last 10 years, over 35% of LLNL’s R&D 100 Awards had roots in the LDRD Program.
Single-Year Statistics | Multi-Year Statistics | |||||
---|---|---|---|---|---|---|
History of R&D 100 Awards at LLNL | FY20 | FY21 | FY22 | FY13–17 (5 yrs) |
FY18–22 (5 yrs) |
FY13–22 (10 yrs) |
Total R&D 100 Awards | 1 | 3 | 3 | 22 | 11 | 33 |
Awards with LDRD roots | 0 | 0 | 2 | 8 | 4 | 12 |
% with LDRD roots | 0% | 0% | 67% | 36% | 36% | 36% |
Average years from 1st LDRD experience | N/A | N/A | 7.5 | 6.9 | 9.8 | 7.8 |
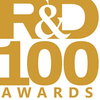
The renowned R&D 100 competition, now in its 60th year, received entries from 17 countries and regions around the world.
Two LDRD-Funded Projects Win R&D 100 Awards in 2022
Two of LLNL’s three R&D 100 Awards – for Energy Inks and Tailored Glass by Direct Ink Writing – received internal funding from the Laboratory Directed Research and Development (LDRD) Program.
The Laboratory has now collected a total of 176 R&D 100 awards since 1978. Energy Inks and Tailored Glass by Direct Ink Writing — received internal “seed money” from the Laboratory Directed Research and Development (LDRD) program. This funding enables undertaking high-risk, potentially high-payoff projects at the forefront of science and technology.
This year’s LLNL R&D 100 awards include an additive manufacturing process called Tailored Glass by Direct Ink Writing that prints silica-based optics and glass components into customizable forms, novel compression gratings that enable a new class of high-energy laser systems, and a three-dimensional printing feedstock known as Energy Inks that can print a functioning battery.
"It is a great tribute to the innovative spirit of our scientists and engineers to be selected for an R&D 100 award," Lab Director Kim Budil said. "Teaming with industrial collaborators is an important element in ensuring that the transformative technologies developed at LLNL will benefit the nation."
The Tailored Glass team is led by chemical engineer Rebecca Dylla-Spears and includes physicists Du Nguyen and Michael Johnson; materials scientists Jungmin Ha, Timothy Yee and Becca Walton; chemists Koroush Sasan and Tyler Fears; chemical engineer Nikola Dudukovic; mechanical engineer Megan Ellis; and optical engineer Oscar Herrera.
Lab scientists and engineers have developed Energy Inks, a three-dimensional (3D) printer feedstock that allows the production of a functioning battery and other devices. 3D printing with polymers allows a newer and more efficient method of prototyping. Now Energy Inks, which have functional properties, are optimized to enable next-generation, high-performance 3D-printed devices for energy storage, catalysis, filtration, sensors, and more uses.
The LLNL team that developed Energy Inks received funding through a Department of Energy Technology Commercialization Fund grant. It is led by materials scientist Swetha Chandrasekaran and chemical engineer Marcus Worsley.
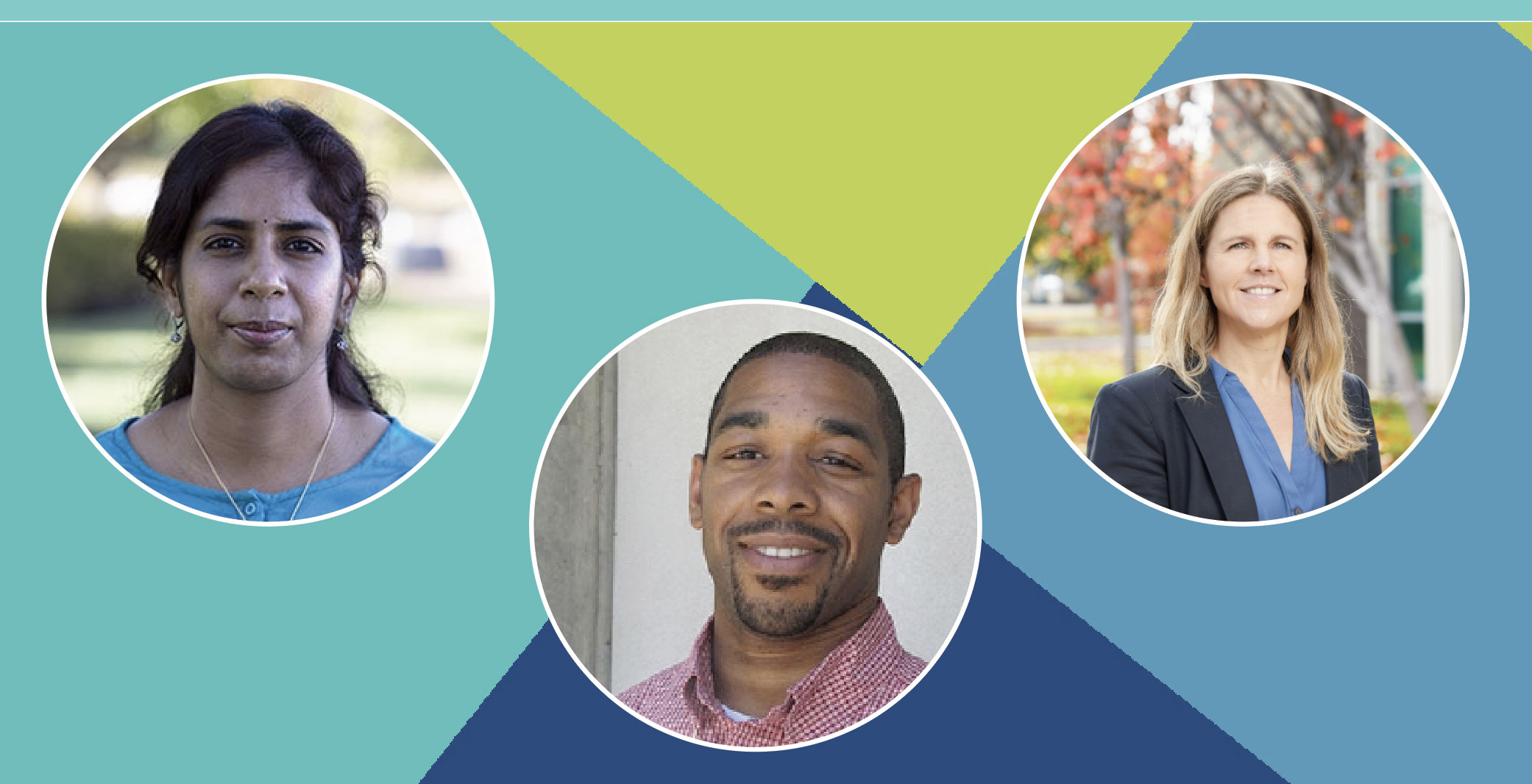